植物生态化学计量特征及其主要假说
Review on characteristics and main hypotheses of plant ecological stoichiometry
通讯作者: **jyfang@urban.pku.edu.cn
编委: 刘玲莉
责任编辑: 谢巍
收稿日期: 2020-10-10 接受日期: 2021-01-7
基金资助: |
|
Corresponding authors: **jyfang@urban.pku.edu.cn
Received: 2020-10-10 Accepted: 2021-01-7
Fund supported: |
|
作者简介 About authors
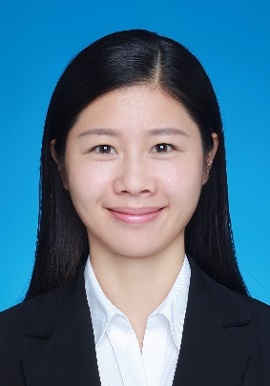
*田地,北京林业大学青年研究员,硕士研究生导师,主要从事植物生态化学计量学和全球变化生态学等方面的研究,研究兴趣在于探索陆生植物不同器官间的化学计量关系、植物功能属性的生物地理格局及成因、陆地生态系统结构与功能对环境变化的响应及预测。在NationalScienceReview、Ecology、EnvironmentalPollution等刊物发表论文35篇,获专利2项,出版译著1部。主持国家级和省部级科研项目共6项。E-mail:
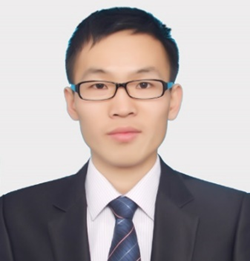
严正兵,香港大学博士后,主要从事植物生态化学计量学、植物功能性状、植物光谱学等方面的研究。在EcologyLetters、NewPhytologist、FunctionalEcology等刊物发表论文40余篇,被引用950余次。主持国家级和香港地区科研项目共4项。现任《草业科学》期刊青年编委,并任Diversity专刊编辑。 。
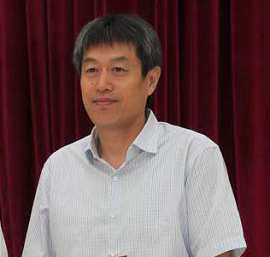
方精云,北京大学教授、云南大学校长、中国科学院院士。主要从事全球变化生态学、植被生态与生物多样性、生态遥感、生态草牧业等方面的研究。发表论文350余篇,被引用4万余次。曾获国家自然科学奖二等奖、长江学者成就奖、教育部及北京市自然科学一等奖以及惠泰克杰出生态学家奖等奖项。 E-mail:jyfang@urban.pku.edu.cn。
植物生态化学计量学是生态化学计量学的重要分支, 主要研究植物器官元素含量的计量特征, 以及它们与环境因子、生态系统功能之间的关系。19世纪, 化学家们通过室内实验, 分析了植物器官的元素含量, 开始了对植物化学元素之间关系的探索。如今, 生态学家通过野外采样和控制实验, 探索植物化学元素计量特征的变化规律、对全球变化的响应以及与植物功能属性之间的关系, 促进了植物生态化学计量学的快速发展。该文在概述植物生态化学计量学发展简史的基础上, 综述了19世纪以来该领域的研究进展。首先, 该文将植物生态化学计量学的发展历程概括为思想萌芽期、假说奠基期和理论构建期3个时期, 对各个时期的主要研究进行了简要回顾和梳理。第二, 概述了植物主要器官的化学计量特征, 尤其是陆生植物叶片氮(N)和磷(P)的计量特征。总体上, 全球陆生植物叶片N、P含量和N:P (质量比)的几何平均值分别为18.74 mg∙g-1、1.21 mg∙g-1和15.55 (与16:1的Redfield比一致); 在物种或群落水平上, 叶片N和P含量一般呈现随温度升高、降水增加而降低的趋势。不同生活型植物叶片N和P计量特征差异明显, 尤其是草本植物叶片N和P含量高于木本植物, 落叶阔叶木本植物叶片N和P含量高于常绿木本植物。与叶片相比, 细根和其他器官化学计量特征研究较少。第三, 总结了养分添加实验对植物化学元素计量特征的影响。总体上, N添加一般会提高土壤N的可利用性, 使植物器官中N含量和N:P升高, 在一定程度上提高植物生产力; P添加可能会缓解过量N输入导致的N-P失衡问题, 提高植物器官P含量。但是, 长期过量施肥会打破植物器官原有的元素间计量关系, 导致元素计量关系失衡和生产力下降。第四, 梳理总结了植物生态化学计量学的重要理论、观点和假说, 主要包括刻画化学计量特征与植物生长功能关系的功能关联假说、刻画化学计量特征与环境因子关系的环境关联假说或理论以及刻画化学计量特征与植物进化历史关系的进化关联假说。最后, 指出了植物生态化学计量学研究中存在的问题, 展望了10个未来需要重点关注的研究方向。
关键词:
Plant ecological stoichiometry, as a branch of ecological stoichiometry, focuses on the study of elemental content, ratios and relationships within and across plant organs, and the underlying biotic and abiotic drivers. In the 19th century, chemists detected the elemental contents in plant organs via laboratory experiments, sprouting the exploration of plant stoichiometric characteristics. Nowadays, ecologists have explored plant ecological stoichiometric characteristics and their responses to global changes and relationships with plant functional traits, using both field investigation and manipulative experiments. These sustained efforts have largely enriched the knowledge and understanding of plant ecological stoichiometry. In this paper, we briefly introduced the history and reviewed the research progresses of plant stoichiometry since the 19th century. Firstly, we proposed the developmental history of plant ecological stoichiometry as three main periods: sprouting, hypothesis foundation, and theoretical construction periods, and introduced some representative works for each period. Secondly, we overviewed plant ecological stoichiometric characteristics across organs, life forms and environmental gradients. The geometric mean values of leaf nitrogen (N) and phosphorus (P) contents and N:P mass ratios in global terrestrial plants are 18.74 mg∙g-1, 1.21 mg∙g-1 and 15.55 (i.e. similar to the Redfield ratio of 16:1), respectively. Leaf N and P contents at either species or community level generally show a decreasing trend with increasing temperature and precipitation, and have large variations among life forms, with higher values in herbaceous than woody plants, and deciduous broad-leaved than evergreen broad-leaved and coniferous woody plants. Compared with leaves, the stoichiometric characteristics of fine roots and other organs in plants remain poorly documented. Thirdly, we reviewed the effects of nutrient addition on plant ecological stoichiometric characteristics. In general, N addition increases soil N availability, then the N content and N:P in plants, thus leading to an increase in plant productivity to some extents. P addition might alleviate the N and P imbalance induced by excessive N inputs, and then increase plant P content. However, long-term nutrient fertilization could perturb the intrinsic stoichiometric characteristics in plants, resulting in the deteriorated nutrient imbalance in tissues and then the subsequent decline in plant productivity. Fourthly, we introduced the main hypotheses of plant ecological stoichiometry. These hypotheses include function-associated hypotheses, environment-associated hypotheses and evolution-associated hypotheses, which delineate the relationships of stoichiometric characteristics with plant growth functions, environmental factors and plant evolutionary history, respectively. Finally, we made an outlook on future research in the area of plant ecological stoichiometry, and highlighted ten potential and important research themes.
Keywords:
引用本文
田地, 严正兵, 方精云.
TIAN Di, YAN Zheng-Bing, FANG Jing-Yun.
作为植物学、化学、物理学的交叉学科, 植物生态化学计量学主要研究植物器官内化学元素的含量及比值等计量特征, 以及它们沿环境梯度变化的规律, 与环境因子、植物个体生长发育、群落动态和生态系统功能等之间的关系(Sterner & Elser, 2002)。植物生态化学计量学研究涵盖了分子、细胞、器官、个体(物种)、群落、生态系统、区域和全球等不同尺度(程滨等, 2010; 田地等, 2018), 是联系微观—宏观生态学研究的重要桥梁(Elser, 2006)。它为植物个体到生态系统统一理论的构建提供了新的视角(贺金生和韩兴国, 2010), 对于理解植物生理生态过程、生物地球化学循环过程、生态系统功能等具有重要的理论意义, 同时对于判断植物体和群落的养分限制状况、指导生态系统养分管理、预测全球养分变化背景下的植被动态等, 也具有重要的现实意义。
近30年来植物生态化学计量学研究蓬勃发展, 尤其在我国, 研究人员通过大量的采样和分析, 获得了一大批植物器官元素含量数据, 产出了一系列重要成果。但同时也存在一些问题。比如, 部分研究有“扎堆”现象; 受采样方法的限制, 较多的研究都关注叶片, 特别是幼苗或林下植物叶片的碳(C)、氮(N)、磷(P)计量特征, 对较难获取的乔木冠层叶片、细根的元素计量特征关注较少; 一些研究停留在对植物生态化学计量特征的描述上, 缺乏机理的探讨。本文将在回顾植物生态化学计量学发展简史的基础上, 梳理并总结近些年来植物生态化学计量领域重要的成果和假说, 并探讨植物生态化学计量学发展中存在的问题及未来的发展方向, 以期为深化植物生态化学计量学研究和理论发展、促进植物生态化学计量理论与应用相结合, 提供一些参考和建议。
1 植物生态化学计量学发展简史
植物生态化学计量学是生态化学计量学的重要内容, 根据其发展过程与阶段性成果, 可将其发展历史大致划分为3个时期: 思想萌芽期、假说奠基期和理论构建期。
1.1 思想萌芽期
生态化学计量学朴素思想的萌芽可以追溯到19世纪40年代。例如, 德国化学家Justus von Liebig (1803-1873)在其研究中发现, 供应量最低的元素决定着植物的生长, 即著名的“李比希最小因子定律” (Liebig, 1843)。之后, 这种限制性元素含量和植物生长密切联系的思想促进了植物营养学和农学的发展, 并延伸至植物生理学、生物种群和生态系统模型等领域的研究中。
19世纪末20世纪初, 欧美国家农化领域的研究人员, 通过化学实验, 分析了草本植物、农作物、果树、森林群落中植物不同器官的元素组成, 尤其关注了植物在不同生长阶段的元素组成变化和元素的潜在功能, 并从经验的角度推测土壤养分供给与植物元素组成之间的可能关系(Serex, 1916; McHargue & Roy, 1932; Leyton, 1957)。总体而言, 这些研究多集中在农林和化学领域, 主要是从解释现实生产、生活中一些现象的角度出发, 关注陆生植物器官(尤其是农作物的叶片)内不同元素含量、元素在器官间的转移、植物生长随个体发育和外界环境的变化规律, 以及养分亏缺对植物生理及表型的影响(Williams, 1955; Bradshaw et al., 1960), 为近现代农林领域测土配方施肥奠定了基础。
相较于陆生生态系统, 这一时期水生生态系统的生物化学循环、生物元素组成及其含量等内容在湖沼学和海洋生态学的发展中逐渐受到关注, 水生植物对元素“奢侈吸收”的现象也较早地被发现(Harper & Daniel, 1934; Cooper, 1937)。20世纪初, 欧洲生态学家认识到湖泊N、P、钙(Ca)等元素的供应与浮游植物数量及其组成之间的关系(Kalff & Downing, 2016)。特别是, 英国植物生态学家William Harold Pearsall在1928-1932年探讨了英国主要湖泊浮游生物的周期性分布与湖水营养物质含量之间的关系, 提出利用水体中可溶性硝酸盐含量和可溶性磷酸盐含量的比值(nitrate:phosphate)作为指示湖泊N或P限制和浮游植物类群周期性分布的指标(Pearsall, 1932)。到20世纪30-50年代, 美国海洋学家Alfred Redfield通过对大西洋、太平洋和印度洋表层海水的多次采样分析, 发现海洋浮游生物的C:N:P平均值约为106:16:1 (摩尔比), 且该值与海洋表层水体中溶解的有机质、N、P的比值存在一致性(Redfield, 1960), 即著名的Redfield比。这从真正意义上拉开了生态化学计量学研究的帷幕, 促进了植物生态化学计量学研究的兴起。
1.2 假说奠基期
20世纪后半叶, 以欧美国家的研究人员为主, 开展了对植物营养元素调查与分析的大量案例研究。这一时期的植物生态化学计量学研究受土壤农化分析思路的影响依然较大, 在分析不同物种的元素组成与含量、诊断生态系统中植物生长的限制性元素、研究贫瘠营养环境中植物的适应性表型特征与生理性策略、通过施肥措施提高植物的生产力等研究中, 提出了“相对生长速率”、“氮磷限制”、“养分重吸收”等概念, 为生态化学计量学理论构建奠定了基础。该时期植物生态化学计量学研究的科学问题大致概括为以下3个方面:
(1) 植物器官内元素组成和含量与环境条件之间的关系。研究对象几乎涵盖了海洋、湖泊、湿地、森林、草地和农田等多种生态系统中的大部分常见物种, 而且这些研究主要是针对某些特定地区而开展的本底性工作。得益于该时期湖沼学和海洋生态学等相关学科在欧洲和美国的迅速发展, 欧美学者对内陆湖泊、入海口、近海、远洋等不同水生生态系统中的浮游植物和大型水生植物进行了大量采样分析, 尤其对常见的眼子菜(Potamogeton sp.)、狐尾藻(Myriophyllum sp.)、金鱼藻(Ceratophyllum demersum)、萍蓬草(Nuphar lutea)、芦苇(Phragmites communis)、睡莲(Nymphaea tuberosa)、虉草(Phalaris arundinacea)等物种的器官元素组成与含量的调查已经比较清楚(如: Allen & Pearsall, 1963; Riemer & Toth, 1969; Boyd & Vickers, 1971; Ho, 1979a; Smart, 1980)。水生植物器官元素含量的比值与环境元素含量(如水生植物/底泥/水体)之间的关系(如: Gerloff & Krombholz, 1966; Ho, 1979b; Jackson et al., 1991; Fong et al., 1998), 及其对植物个体生长速率、生态系统中生产者-消费者之间的相互作用、生态系统生产力、水环境质量(富营养化)的影响(Smith, 1982; Tett et al., 1985; Horrocks et al., 1995; Hassett et al., 1997), 是探讨较多的问题; 而且许多研究检验了水生植物C:N:P计量比与Redfield比值的异同(Elser et al., 2000; Sterner & Elser, 2002; Yan et al., 2016c)。
陆生生态系统中植物生态化学计量特征的研究对象包括实验室/温室栽培的幼苗和自然环境中的野生植物(如: Ingestad, 1962; Woodwell et al., 1975; Chapin III, 1980a, 1980b; Thompson et al., 1997), 其中自然环境中的野生物种较多地集中在温带落叶木本植物、针叶树种、地中海常绿硬叶树种以及多种草本植物(如北极苔原带的多年生草本植物)等。这些物种通常是地域性的优势物种, 或者代表着本地区独特的群落组成, 对其元素组成及含量的分析多与该地区典型的生态过程或生态现象联系起来。例如, 在阿拉斯加苔原带, 常见由白毛羊胡子草(Eriophorum vaginatum)构成的草丛。相较于草丛外部, 草丛内融雪较快, 土壤温度回升使得其生长季相对较长, 加上土壤可利用养分含量较高, 白毛羊胡子草浅层根系繁茂, 较强的营养吸收能力促进生物量的快速累积, 发展成其独特的形态和生理特征, 并形成了独特的地域性景观(Chapin III et al., 1979)。常绿物种通常被认为是植物对贫瘠环境的适应(Chapin III, 1980a)。在荷兰欧石南(Erica)湿性荒原, 通过对常绿矮小灌木Erica tetralix和多年生草本植物天蓝麦氏草(Molinia caerulea)器官元素含量和地上部分生物量进行对比分析, 发现当环境养分可利用性升高时, 该地区原先由E. tetralix占优势的湿性常绿灌丛群落转变为由天蓝麦氏草占优势的落叶草本群落(Aerts & Berendse, 1989)。
(2) 不同功能群植物器官元素计量特征的差异及可能机制。水生生态系统中的研究大多关注水体营养元素变化(富营养化、施肥、排污等)与藻类N和P计量特征及其生物量间的关系(如: Ryther & Dunstan, 1971; Goldman et al., 1979; Rhee & Gotham, 1980; Fong et al., 1998)。陆生植物的研究多与植物生理密切联系。较早的研究多集中在个体或物种水平, 采用幼苗营养液培养实验或温室幼苗施肥控制实验, 探讨养分供应—植物器官元素含量—植物生长发育之间的关系, 明确不同元素对植物生理过程的调控作用(Chapin III, 1980b; Ågren, 1988; Marschner et al., 1997), 以期找到有利于植物生长的最佳养分配比(如: Ingestad, 1962, 1970, 1971, 1979; van den Driessche, 1974; Aronsson & Elowson, 1980)。随着自然生态系统中大范围野外群落调查和施肥实验的开展, 研究人员开始在群落和生态系统水平上, 分析不同生活型植物的化学计量特征, 及其与环境养分可利用性、植物其他生理生态属性间的关系(如: van Andel & Vera, 1977; Chapin III & Kedrowski, 1983; Körner, 1989; Aerts & Chapin III, 1999), 尤其是土壤养分供应与植物养分吸收、养分重吸收对植物生态化学计量特征的影响成为该时期的重要研究领域(Chapin III, 1980a; Shaver & Melillo, 1984; Killingbeck, 1986)。
(3) 生长速率与氮磷限制问题。有机体的生活史特征(尤其是生长速率)与其生化组分和N-P计量特征密切相关。从生化的角度分析, 主导细胞与有机体N-P计量特征的分子是蛋白质和核酸, 因为蛋白质含N量高且对生物量贡献大, 而核酸的N和P含量均较高且相对丰度较高, 所以有机体的细胞结构和整个有机体N-P计量特征本质上的决定因素是蛋白质和核酸相对丰度的比值。理论上来说, 有机体较高的生长速率需要大量核糖体和蛋白质合成来维持, 因此其细胞具有较多的核糖体核糖核酸(rRNA), 也相应地具有较高的P含量和较低的N:P; 反之, 生长较慢的有机体, 其P含量相对较低, N:P较高(Sterner & Elser, 2002)。这便是基于N-P计量比的“相对生长速率”假说的核心内容(Elser et al., 1996), 该假说在这一研究时期得到了初步的验证。例如, 对英格兰中部常见草本植物和矮灌木的叶片养分含量进行检测发现, 59种植物叶片的P含量与其最大潜在相对生长速率显著正相关(Thompson et al., 1997)。植物生长速率与生境中的养分可利用性密切关联(Aerts & Chapin III, 1999)。当植物在其最佳条件下生长时, 来自土壤肥沃生境的植物物种比来自贫瘠环境的物种具有更高的相对生长速率(Lambers & Poorter, 1992)。因此, 在具体的生态系统中, 植物生长是否存在养分限制, 或者说环境营养元素是否可以充分支持植物实现最大速率生长, 成为这一研究时期探讨较多的问题。由于养分限制的问题最初来自农业, 传统上判定养分限制的方法是开展施肥试验。Koerselman和Meuleman (1996)较早地提出利用植被的N:P作为指示群落水平上N限制或P限制的方法(截至目前, 该文在Web of Science上的被引用次数达1 574次)。在此之后, 判断生态系统养分限制的研究成为水生植物和陆生植物化学计量学研究中的一种“潮流”, 也成为很多研究的主体思路。
1.3 理论构建期
2000年挪威科学院就生态化学计量学召开了会议, 来自林学、海洋学、昆虫生物学、生态学和土壤生物学等领域的20多位科学家参会, 提出了一个合作研究计划, 即检验在水生生态系统中得到的关于生态化学计量学的经验性和理论性成果在陆地生态系统中是否也存在普遍性(Elser, 2000)。之后, 全球范围内的植物生态化学计量研究蓬勃发展, 尤其是陆生植物N-P计量特征研究积累了大量数据, 使得大尺度上植物生态化学计量特征随环境梯度的变化格局、全球变化因子对植物生态化学计量特征的影响、植物生态化学计量特征与其他功能属性及生态系统过程、结构和功能的关系, 成为植物生态化学计量学研究的主要内容。
该时期, “生长速率假说”、“N:P阈值假说”、“相对重吸收假说”得到更多研究的验证与发展。同时, 用于解释大尺度上植物N-P计量特征变化格局的“温度-植物生理假说”、“生物地球化学生态位假说”、“物种组成假说”、“土壤基质年龄假说”、“限制元素稳定性假说”、“化学计量内稳性理论”等不断完善(Sterner & Elser, 2002; Reich & Oleksyn, 2004; Han et al., 2005, 2011; Sistla & Schimel, 2012), 构成了植物生态化学计量特征驱动机制的理论体系。另外, 在应用层面, 植物生态化学计量特征与个体生长速率、个体大小、能量代谢等功能属性之间关系的理论和模型不断发展(Ågren, 2004; Niklas et al., 2005; Elser et al., 2010; Sardans et al., 2012), 对阐明生态系统结构、功能和过程产生较大贡献; “叶经济谱属性”理论在植物生态化学计量特征与叶片的结构-生理特性之间构建了关联(Wright et al., 2004), 为大尺度养分循环和植被动态预测提供了重要工具。
这一时期另一个突出的特点是: 我国植物生态化学计量学研究突飞猛进, 成为国际上生态化学计量学研究的重要力量。1995年, 方精云等在北极科考时发现北极冰、雪和冻土的元素含量之间存在极好的相关性(方精云和刘少创, 1999; 殷兴军和方精云, 1999; 方精云, 2000), 启发了其研究团队对陆生植物生态化学计量特征的研究, 提出了多个观点或假说。例如, 其团队定量分析了11种陆生植物化学元素的计量特征、大尺度地理格局及其生态成因, 提出“限制元素稳定性假说” (Han et al., 2005, 2011); 将植物养分的大尺度化学计量特征研究由个体以及单个器官扩展到群落层次, 并探索了群落水平植物不同器官C、N、P化学计量特征及其与生态系统初级生产力的关系(Tang et al., 2018); 构建了全球最大的植物叶片N和P含量匹配数据库(Tian et al., 2019a), 并从多个尺度上证明陆生植物叶片N和P含量的幂指数关系并非恒定的观点(Tian et al., 2018b, 2019b); 通过野外N和P添加实验和室内实验搭建养分添加对植物生态化学计量特征影响的研究平台, 提出“养分含量-个体变异”等假说(如: Yan et al., 2015, 2016a, 2018, 2019)。另外, 于贵瑞的研究团队以中国东部森林生态系统为对象, 测定了群落内所有植物种类(约1 176种)的叶-枝-干-根中多元素的含量(任书杰等, 2007; 何念鹏等, 2018)。通过这种大规模的采样与分析, 研究人员尝试建立植物个体和群落水平上的化学计量特征与宏观尺度上生态系统功能性状间的定量关系, 发展了生态系统功能性状理论(Zhao et al., 2016, 2020; Zhang JH et al., 2018; He NP et al., 2019, 2020)。
在草地生态系统中, 韩兴国团队较早地对我国草原植物的C、N含量及N的重吸收效率开展了研究(苏波等, 2000; 袁志友等, 2004; Yuan et al., 2005), 综述了植物N:P计量比在生态学应用中的前景及缺陷(Zhang et al., 2003), 并关注了N、P添加对草地植物生态化学计量特征的影响及其可能产生的生态效应。例如, 研究人员将内蒙古锡林郭勒草原进行的N和P添加实验与长期监测相结合, 从时间和空间尺度证明, 植物生态化学计量内稳性是草地生态系统结构、功能和稳定性维持的重要机制(Yu et al., 2010)。贺金生团队在植物生态化学计量特征与群落动态、生产力、生物地球化学循环等关系的研究方面也积累了大量数据(He et al., 2010; Geng et al., 2017), 探索了我国主要草原植物叶片C、N、P计量特征的大尺度格局, 检验了草原植物叶片化学计量特征与其他叶属性间的关系是否符合陆生植物的一般规律等科学问题(He et al., 2006a, 2006b, 2008)。
除此之外, 一些局部地区或针对特定类群的调查研究, 在探讨植物生态化学计量特征与生物因子和非生物因子之间的关系, 尤其是对全球变化的响应方面, 也获得了一些重要的数据和结论(如: Wu et al., 2012; Yang et al., 2015; Sun et al., 2016; Tao et al., 2016; 蔡琴等, 2019; Luo et al., 2020)。例如, 何明珠团队(He et al., 2015, 2016a, 2016b)和Luo等(2021)对干旱半干旱区荒漠植物不同器官的C、N、P计量特征及其与气候、土壤养分可利用性、植物生活型之间的关系进行了较为细致的研究, 尤其关注干旱对植物生态化学计量关系的影响(He & Dijkstra, 2014; He et al., 2014; Zhang et al., 2019)。
2 植物主要器官的化学计量特征及其环境影响
2.1 叶片氮磷计量特征
叶片是植物光合作用的重要器官。以往研究发现, 叶片N含量与光合速率、呼吸速率和生产力之间存在正相关关系(Evans, 1989; Wright et al., 2004; Reich et al., 2009), 叶片N、P含量之间存在强烈的耦合关系(Thompson et al., 1997; Reich et al., 2010), 叶片P含量调控植物生理过程(尤其是生长速率)和叶片N-生产力之间的关系(Niklas, 2006; Reich et al., 2009); 而且, 叶片N和P计量特征也被广泛用于评估植物/生态系统养分限制(Güsewell, 2004)和大尺度植被生产力的模型估算(Smith et al., 2002)。因此, 植物叶片N、P含量及其计量特征备受关注。
经统计发现, 全球陆生植物叶片N、P含量和N:P质量比的几何平均值分别为18.74 mg∙g-1、1.21 mg∙g-1和15.55, N和P计量特征在不同个体、物种、生活型、生境、温度带等分类水平上存在较大的差异(表1), 其中个体间叶片N含量可相差30倍, P含量的差异可达到100倍以上, N:P变动范围约在1-100之间(Tian et al., 2018b)。在物种或群落水平上, 植物叶片N和P含量的分布格局一般呈现随年平均气温和降水量的增加而降低的趋势(Reich & Oleksyn, 2004; Tang et al., 2018; 图1)。在不同生活型间, 快速生长、生活史较短的草本植物叶片N和P含量较高, 生长速率缓慢、生活史较长的常绿植物叶片N和P含量较低, 落叶物种居于中间(Han et al., 2005; Chen et al., 2013; Tian et al., 2019b)。但是, 前人的研究结果存在差异。例如, 中国植物叶片P含量低于全球平均水平(Han et al., 2005; 任书杰等, 2007), 原因可能在于中国土壤P含量较低; 某些科属植物, 如蒿属(Artemisia), N-P计量特征受温度和降水的影响较弱, 未呈现明显的纬度变异格局, 但却呈现出沿海拔梯度变化的格局(Yang et al., 2015), 这可能是局部地区采样中温度和降水梯度不明显导致的结果。
表1 全球陆生植物叶片氮(N)和磷(P)含量和N:P在不同生活型间的差异
Table 1
功能群 Functional group | N (mg∙g-1) | P (mg∙g-1) | N:P (g∙g-1) | n | |
---|---|---|---|---|---|
所有生活型 All functional groups | 18.74 (±8.33) | 1.21 (±0.94) | 15.55 (±9.95) | 12 721 | |
所有木本 All woody species | 18.22 (±7.77) | 1.10 (±0.81) | 16.53 (±9.30) | 9 098 | |
常绿针叶 Evergreen conifers | 12.79 (±4.25) | 1.06 (±0.81) | 12.07 (±8.39) | 480 | |
常绿阔叶 Evegreen broadleaved | 15.29 (±6.66) | 0.79 (±0.56) | 19.29 (±10.60) | 3 476 | |
落叶阔叶 Deciduous broadleaved | 21.29 (±7.53) | 1.40 (±0.86) | 15.24 (±7.66) | 4 981 | |
其他木本 Other woody species | 18.63 (±8.32) | 1.23 (±0.64) | 16.58 (±8.77) | 101 | |
草本 Herbaceous species | 20.56 (±9.33) | 1.56 (±1.12) | 13.17 (±11.03) | 3 489 | |
蕨 Ferns | 11.46 (±4.75) | 0.65 (±0.62) | 17.75 (±13.06) | 134 |
数据来自Tian等(
Data were cited from Tian et al. (
图1
植物叶片N、P含量和N:P计量比为什么会呈现出明显的环境梯度变化规律? 什么因素决定了或者说如何塑造了这样的变化规律? 研究人员对此提出了多种推测。例如, 不同物种的养分吸收能力决定了其叶片养分含量(Osone & Tateno, 2005); 植物生理特征及过程调控着叶片N、P计量特征(如奢侈吸收、重吸收、稀释效应、叶寿命变化、个体大小等) (Eckstein et al., 1999; Ågren, 2008; Sardans & Peñuelas, 2014); 气候条件和土壤发育阶段影响植物叶片N、P计量特征(Parfitt et al., 2005; Sardans et al., 2011)。总结起来, 以往研究中对叶片N、P计量特征的归因可以概括为两方面: (1)与物种特性相关的系统发育/遗传因素; (2)环境因素, 包括气候和土壤条件等。但是, 如何区分自然选择/系统发育/遗传因素和环境因素对叶片N和P计量特征的影响? 有研究指出, 植物养分含量可能存在系统发育信号(Thompson et al., 1997; Peñuelas et al., 2010; Hao et al., 2015; Sardans et al., 2015); 但也有研究表明, 在系统发育上亲缘关系较近的物种, 其化学计量特征也常常存在较大变化, 而温度和降水等环境条件可能比系统发育更直接地或在更大程度上影响叶片化学计量特征的空间格局(Ågren & Weih, 2012; Zhang et al., 2012; Yang et al., 2015)。总之, 理解叶片N和P计量特征环境格局形成的机制, 十分关键但也比较困难, 是未来值得探索的重要问题。
除了N、P含量和N:P外, 叶片N和P计量特征也可以定量化地表示为N = βPα (简称为N-P幂函数关系), 其中α和β分别指对数变换后叶片N含量相对于P含量做主轴回归的斜率和截距(McGroddy et al., 2004; Niklas et al., 2005; Kerkhoff et al., 2006)。下文将详细阐述叶片N-P计量关系的幂函数是否守恒的问题(Reich et al., 2010; Tian et al., 2018b)。目前来看, 虽然全球陆生植物叶片N-P幂函数关系的总体格局已经清楚, 叶片N-P计量幂指数存在较大差异, 但其变化的驱动机制是什么? 而且, 叶片N-P幂函数关系是基于种群、群落或生态系统水平上多样本混合统计分析得出的, 从本质上而言, 样本量大小和样本来源/组成如何影响N-P幂函数关系? 这些问题目前都尚无答案。
2.2 细根氮磷计量特征
细根是陆地生态系统生物地球化学循环中重要的养分来源和库。从20世纪90年代开始, 研究人员开始关注地下部分根系的养分含量。Jackson等(1997)首次阐述了全球细根(直径≤2 mm)生物量、长度和表面积随土壤深度的分布格局, 以及细根养分库和计量比的全球估算。结果显示, 活细根的平均C:N:P质量比为450:11:1, 全球细根C库的总量是大气C库的5%以上; 与地上部分树木生物量相比, 细根的直径较小, 寿命相对较短, C:N较低, 导致它们的周转和分解速度较快, 这使其在估算年净初级生产力、养分循环和C分配中尤为重要。Gordon和Jackson (2000)综合前人的数据, 发现细根养分含量与细根直径负相关, 直径≤2 mm的存活细根平均N和P含量为11.0和0.9 mg∙g-1, 直径在2-5 mm的根系平均N和P含量为6.5和0.6 mg∙g-1; 而且, 存活细根和死亡细根中平均N含量相同, 说明细根在死亡过程中养分转移量很低。利用北方森林研究中可用的根系养分数据, Yuan和Chen (2010)分析了根系N、P计量特征与环境因子的关系: 当所有数据混合在一起时, 根系N含量随着纬度升高而降低, 但是P含量保持稳定; 根系N和P含量均随年平均气温和降水量的升高而降低。之后, Yuan等(2011)通过对不同径级植物细根的分析, 得出全球植物细根平均N和P含量为10.2和0.83 mg∙g-1, 根系N和P含量随着根系径级的增大而降低, N:P升高。相较于存活根系, 死亡根系的C和P含量较低, N含量变化不大。所有径级的存活根系和死根的N:P随纬度升高呈现指数型下降, 而且不同温度带的细根N、P含量和N:P存在显著差异。马玉珠等(2015)对中国植物根系C、N和P数据的分析发现, 中国植物细根C、N、P含量的平均值为473.9、9.2和1.0 mg∙g-1, N:P、C:N和C:P质量比分别为14.27、59.15和522.10; 粗根(直径>2 mm)的C、N、P含量平均值为456.5、5.9和0.6 mg∙g-1, N:P、C:N和C:P质量比分别为11.67、121.98和957.27; 对于细根和粗根, C含量及N:P和C:N随纬度升高而降低, 而P含量随纬度升高而升高; 总体上土壤养分情况在很大程度上影响着根系的N和P含量。
必须指出, 上述研究是基于前人有限数据的整合分析, 而且不同研究中采样方法、根系分级、样本量等差异对结果的影响很难定量化, 使得这些结果具有一定的不确定性。Li等(2010)利用从中国和俄罗斯西伯利亚地区按统一方法采集到的49个物种不同分级根系的元素含量实测数据, 证明了树木根系N和P含量与根系级别之间存在负相关关系, 即细根中养分含量高于主根, 而且毛细根的根尖部位N和P含量及N:P与叶片一致, 同时也发现根系N含量在不同温度带、P含量在不同分类群中均存在明显差异。
表2 关于根系氮磷计量特征研究的文献及主要结果*
Table 2
![]() |
2.3 其他器官氮磷计量特征
相较于叶片和根系, 关于植物其他器官N-P计量特征的研究相对较少, 仅限于一定的区域或某些植物类群。例如, de Frenne等(2011)对欧洲森林广泛分布的草本植物Anemone nemorosa种子养分计量特征的研究发现, 种子N含量和N:P随纬度(北纬)升高而下降, 这与叶片N和P计量特征的纬度格局一致(Reich & Oleksyn, 2004), 但是种子的收获期影响其N和P计量特征。在木本植物中, 树皮N和P含量比木质部高出5倍以上(Harmon et al., 1986); 心材的N和P含量低于边材, 而且裸子植物边材和心材的N和P含量低于被子植物(Meerts, 2002)。中国西部地区荒漠植物红砂(Reaumuria soongarica)的茎和根的N含量低于叶片, 但是P含量和N:P在不同器官间差异并不显著(He et al., 2015)。在中国东部森林, 木本植物茎干的N和P含量随着海拔的升高而降低, N:P升高, 但是N-P幂函数关系没有发生变化(Zhao et al., 2016)。Yan等(2016b)对中国东部12处森林335个木本植物物种当年生小枝(末端10-20 cm处的枝条)的叶片N-P幂函数关系进行研究, 发现随着植物养分含量的增加, 低纬度地区植物的小枝N和P含量增加速度比叶片快, 而高纬度地区植物小枝N和P含量增加速度比叶片慢, 这种从低纬度到高纬度的养分分配策略变化可以通过温度来控制。Tang等(2018)发现在群落水平上, 我国陆生植物茎干N和P含量随年平均气温/年降水量升高呈现下降的趋势(图1)。尽管近些年来, 在全球植物功能属性数据(TRY)库中已经有研究人员贡献了植物不同器官元素含量的数据(Kattge et al., 2011), 也有文献报道了相关的数据(Vayreda et al., 2016), 但总体上关于植物叶片和根系以外其他器官化学计量特征的研究依然很少, 这也在很大程度上限制了我们对植物根叶以外的器官化学计量的认识及其在生态模型中的应用(Chave et al., 2009)。
3 施肥对植物生态化学计量特征的影响
通过施肥提高植物生产力是人类一直以来努力的目标, 尤其是农业生态系统中施肥的成功经验, 也促进了施肥在森林等生态系统中的应用。N和P是陆地和水生生态系统普遍存在的限制元素(Elser et al., 1990, 2007)。通常认为温带地区生态系统(如北方森林、苔原带、温带落叶林、山区草地或灌木林等) N相对缺乏; 而热带和亚热带地区, 以及温带针阔叶混交林、针叶林、荒漠地区、地中海地区、萨瓦纳草原与灌丛等P相对缺乏(Vitousek et al., 2010; Du et al., 2020)。尽管传统观点认为由于固氮藻类等微生物的广泛存在, 水生生态系统通常受到P的限制(Sterner & Elser, 2002), 但基于不同生态系统N和P添加实验的结果证实水生生态系统与陆生生态系统面临类似的N和P限制(Elser et al., 2007)。人类活动导致大量氮氧化物进入大气, 之后通过干沉降和湿沉降的形式, 再次进入陆地和水生生态系统, 可能会改变这些生态系统固有的N和P的循环规律和过程, 进而影响植物生长及生态系统的结构与功能(Galloway et al., 2004; Peñuelas et al., 2012)。N沉降的生态效应是近几十年来全球变化生态学关注的热点问题(Vitousek et al., 1997; Liu et al., 2011)。研究人员常常通过施肥实验来模拟N沉降及其可能产生的生态系统N饱和或P限制问题(Wright & van Breemen, 1995; Du et al., 2013; Song et al., 2019)。
全球N沉降模拟实验平台包括欧洲N饱和实验平台(NITREX)(Wright & van Breemen, 1995), 美国哈佛森林实验(Harvard Forest)(Aber et al., 1993), 鼎湖山亚热带常绿阔叶林N添加实验(Mo et al., 2008), 中国森林养分添加实验(Nutrient Enrichment Experiments in China’s Forests, NEECF)(Du et al., 2013), 鸡公山落叶阔叶混交林林冠模拟N沉降大型实验平台(Zhang et al., 2017), 以及其他养分添加实验(田地, 2017), 关注了N添加对植物N和P计量特征的影响。综合这些实验的结果, 可以发现N添加一般会提高土壤N的可利用性, 尤其是在N相对受限的温带森林中, N添加使得植物器官中N含量升高, 在一定范围内促使生产力提高(图2; Aerts & Chapin III, 1999; Chen et al., 2016; Yue et al., 2017); 但也有可能打破植物器官原有的元素间的计量比, 导致元素关系失衡, 不利于植物生长, 这种情况多出现在长期施N实验或N元素相对充足的热带森林(Mohren et al., 1986; Högberg et al., 2006; Peñuelas et al., 2013; Carnicer et al., 2015)。P添加相应地会提高P的可利用性, 使得植物P含量升高, N:P下降(图2; Yuan & Chen, 2015)。
图2
图2
植物叶片碳(C)、氮(N)、磷(P)计量特征对N和P添加的响应(改自Yuan和Chen (2015))。圆圈表示自然环境中的样本, 灰色和绿色分别表示ln (响应比)与0差异不显著(p > 0.05)和差异显著(p ≤ 0.05); 三角形表示控制环境中的样本, 灰色和红色分别表示ln (响应比)与0差异不显著(p > 0.05)和差异显著(p ≤ 0.05)。误差线显示的是平均值的95%置信区间。括号外和括号内的数字分别代表自然环境和控制环境中的样本量。
Fig. 2
Natural log response ratios of plant carbon (C), nitrogen (N), phosphorus (P) and their stoichiometric ratios to N and P additions, respectively (replotted based on Yuan & Chen (2015)). Circles are for results in natural environments with grey and green representing insignificant (p > 0.05) and significant (p ≤ 0.05) difference between the log response ratio and zero, respectively. Triangles are for results in controlled environments with grey and red representing insignificant (p > 0.05) and significant (p ≤ 0.05) difference between the log response ratio and zero, respectively. Error bars are the 95% confidence intervals for the mean. The numbers out- and inside parentheses represent the numbers of observations for experiments in natural and controlled environments, respectively.
由于不同研究地点的特殊性, 以及不同实验处理之间的差异性, 对这些实验结果的分析常常不具可比性。Sardans等(2012)整合分析了N沉降和N施肥对植物C、N和P计量特征的影响, 发现陆生生态系统中植物和土壤的C:N多呈现下降趋势, 而N:P升高, 尤其是新鲜叶片和凋落物叶片的C:N和N:P呈更显著趋势。Sistla等(2015)对81篇文献中的397项研究进行综述发现: 施N降低了海洋和淡水生态系统中植物的C:N和C:P, 以及水生生态系统中的N:P, 在陆生生态系统中也呈现类似的变化趋势(即N:P呈现下降趋势但不显著), 这些结果与Sardans等(2012)的结论存在部分差异。Tian等(2018a)整合分析了中国施N实验对森林植物器官N和P计量特征的影响, 包括0-480 kg∙hm-2∙a-1不同剂量的N添加。这些实验中绝大部分N添加水平超过了实际N沉降水平, 而且多关注幼苗或林下植物叶片的N和P计量特征, 结果显示不同生活型植物和植物不同器官的N和P计量特征(包括N和P含量、N:P及N-P幂函数关系)呈现差异较大的变化趋势。类似地, 全球其他区域森林生态系统和草地生态系统多个植物器官N和P计量特征对施N的响应结果也存在差异(Ostertag, 2010; Mayor et al., 2014; Sardans et al., 2017)。总体上, 施N对草本植物和林下植物N和P计量特征的影响更加显著(Tian et al., 2017; Zhan et al., 2017); 衰老叶片、茎、根系N和P含量对施N的响应很可能比新鲜叶片更敏感(Schreeg et al., 2014)。
结合外在环境条件和内在生理机制进行综合分析, 可以概括为如下结论: 自然生态系统中植物器官N和P计量特征对施N的差异性响应与生态系统原本的养分可利用性、N和P限制状况以及物种的化学计量内稳性有关(Sistla & Schimel, 2012; Yu et al., 2015; Yue et al., 2017)。例如, 在热带低地森林, 施N没有改变叶片N含量, 但是施P会提高叶片P含量, 降低N:P (Mayor et al., 2014), 这很可能与热带森林生态系统普遍受P限制的特点有关(Deng et al., 2017)。对比不同生活型植物, 在养分相对贫瘠的生境中生长的常绿物种对施肥的响应可能较低(Aerts & Chapin III, 1999), 这可能与常绿植物生态化学计量内稳性, 即在环境条件变化的情况下植物维持其内在元素含量及化学计量关系的能力(Sistla et al., 2015)较高有关。但是, 目前我们对不同物种或不同生活型植物的化学计量内稳性及其如何调控化学计量特征的认识还十分不足。而且, 与叶片相比较, 我们对植物其他器官化学计量特征对N添加响应的关注相对较少。
在室内实验平台上通过调控营养液供给水平, 研究模式植物拟南芥(Arabidopsis thaliana)器官N和P计量特征对施N的响应, 可以避免自然生态系统中N和P限制以及物种间化学计量内稳性差异很难判断和量化的问题。北京大学方精云团队在NEECF平台的基础上(Du et al., 2013), 搭建了在不同N和P添加水平下连续种植多代拟南芥的室内实验平台。种植13代的拟南芥的结果显示: 随着N添加水平的升高, 拟南芥器官内N含量呈现先增加后趋于稳定的趋势, P含量呈现先下降后趋于稳定不变的趋势, 且这种交互影响在拟南芥不同生育期和不同器官间存在明显差异(Yan et al., 2015, 2016a, 2018, 2019; 严正兵, 2017)。对拟南芥化学计量特征的研究结果验证了自然生态系统中的一些结论, 但是长期施N是否会影响植物生态化学计量特征的遗传发育信号, 使植物带有化学计量方面的“记忆特征”, 还有待进一步的验证。
4 植物生态化学计量学的主要理论和假说
植物生态化学计量学主要研究植物体内元素含量和组成及其随生物和非生物因子变化而变化的规律, 目前已有多个理论、观点和假说被提出用来刻画植物生态化学计量学特征。概括而言, 这些理论、观点和假说主要可以分为以下几个方面: (1)功能关联假说, 即刻画化学计量特征与植物生长功能关系的假说, 包含生长速率假说(Sterner & Elser, 2002), N:P阈值假说(Koerselman & Meuleman, 1996; Güsewell, 2004), N-P计量关系的幂指数法则(Niklas et al., 2005; Reich et al., 2010), 生产力-养分分配假说和生产力-叶片寿命假说(Tang et al., 2018); (2)环境关联假说或理论, 即刻画化学计量特征与环境因子之间关系的多种假说, 包含化学计量内稳性理论(Sterner & Elser, 2002), 限制元素稳定性假说(Han et al., 2011), 叶片养分含量稳定假说(Tang et al., 2018), 温度-生物地球化学假说(Reich & Oleksyn, 2004)和温度-植物生理假说(Reich & Oleksyn, 2004), 土壤基质年龄假说(Reich & Oleksyn, 2004)和相对重吸收假说(Han et al., 2013); (3)进化关联假说, 即刻画化学计量特征与植物进化历史关系的假说, 包含物种组成假说(Reich & Oleksyn, 2004), 常绿-落叶假说(Reich & Oleksyn, 2004), 生物地球化学生态位假说(Peñuelas et al., 2008, 2019)(图3; 框1)。下面将围绕这些观点、假说或理论进行阐述。
图3
图3
植物生态化学计量学的主要理论和假说。这些理论和假说主要包含3类: (1)功能关联假说(蓝色标注), (2)环境关联理论和假说(棕色标注), (3)进化关联假说(绿色标注)。图中卡通图和谱系树图于2020年12月10日引自Google图片网站。
Fig. 3
Main theories and hypotheses in plant stoichiometry. Three categaries are included: (1) function-associated hypotheses (annotated in blue), (2) environment-associated hypotheses (annotated in brown), and (3) evolution-associated hypotheses (annotated in green). The cartoon graphs and phylogenetic tree graph are cited from the google image website (
框1 植物生态化学计量主要理论和假说概述
1 功能关联假说
描述化学计量特征与植物生长功能的关联, 主要包括:
(1)生长速率假说(Growth Rate Hypothesis) (Sterner & Elser, 2002): 随生长速率增加, 植物N:P和C:P呈降低趋势, 而P含量呈增加趋势。该假说有助于理解植物生长速率的调控机制, 但受其他因素调控的影响, 在高等植物中并不完全适用。
(2)N:P阈值假说(N:P Threshold Hypothesis) (Koerselman & Meuleman, 1996; Güsewell, 2004): 存在特定N:P阈值来判定植物养分限制状况。该假说为判定植物养分限制类型提供了简易的手段, 但存在很大的不确定性。
(3) N-P计量关系的幂指数法则(Power Law of Leaf N-P Scaling) (Niklas et al., 2005; Reich et al., 2010): N、P含量存在幂函数计量关系, 该法则有助于了解植物N、P含量的耦合关系, 但其幂指数随生物和非生物因子的变化而变化。
(4) 生产力-养分分配假说(Productivity-Nutrient Allocation Hypothesis) (Tang et al., 2018): 群落水平的植被生产力和叶片养分含量间的关系受器官间养分含量的调控, 且在不同生活型间存在差异。
(5) 生产力-叶片寿命假说(Productivity-Leaf Lifespan Hypothesis) (Tang et al., 2018): 群落水平的植被生产力和叶片养分含量关系受叶片寿命的调控, 在落叶植物和常绿植物间存在差异。假说(4)和(5)有助于理解植被生产力的调控机制和群落组成的维持机制。
2 环境关联理论和假说
刻画植物生态化学计量特征与环境因子的关系, 主要包括:
(1) 化学计量内稳性理论(Stoichiometric Homeostasis Theory) (Sterner & Elser, 2002): 植物在变化的环境中具有保持体内养分组成相对稳定的能力。该假说有助于理解植物对外界环境的适应能力。
(2) 限制元素稳定性假说(Stability of Limiting Elements Hypothesis) (Han et al., 2011): 由于生理和养分平衡的制约, 限制性更大的元素在植物体内的含量具有较高的稳定性, 其对环境变化的响应也较为平稳。
(3)叶片养分含量稳定假说(Stable Leaf Nutrient Content Hypothesis) (Tang et al., 2018): 叶片养分含量相比根、茎养分含量更稳定, 对外界环境条件的响应程度更低; 说明叶片以外的其他器官也许能更准确地用于判定植物养分限制状况。
(4)温度-生物地球化学假说(Temperature-Biogeochemistry Hypothesis) (Reich & Oleksyn, 2004): 温度影响着土壤微生物的活性, 从而影响着土壤N和P的可利用性, 改变植物叶片N、P计量特征。
(5) 温度-植物生理假说(Temperature-Plant Physiology Hypothesis) (Reich & Oleksyn, 2004): 植物生长代谢速率受温度调控, 低温条件下植物需要更高的N和P含量, 以补偿生理效率的降低。
(6) 土壤基质年龄假说(Soil Substrate Age Hypothesis) (Reich & Oleksyn, 2004): 土壤发育年龄影响土壤母质的养分供给能力, 进而影响植物养分计量特征。
假说(4)、(5)和(6)在解释植物化学计量的纬度格局的调控机制中适用, 但也存在不确定性。
(7)相对重吸收假说(Relative Resorption Hypothesis) (Han et al., 2013): 植物在落叶前倾向于吸收更多比例的限制性元素, N、P的相对重吸收效率与养分限制类型密切相关, 当N重吸收效率大于P重吸收效率时, 指示该植物可能为N限制, 反之亦然。该假说有助于理解植物的养分利用和循环过程, 判定养分限制类型。
3 进化关联假说
描述化学计量特征与植物进化历史的关系, 主要包括:
(1) 物种组成假说(Species Composition Hypothesis) (Reich & Oleksyn, 2004): 物种或生活型组成的差异影响植物叶片化学计量特征的生物地理格局。
(2) 常绿-落叶假说(Evergreen-Deciduous Hypothesis) (Reich & Oleksyn, 2004): 常绿植物和落叶植物因叶片寿命的差异, 导致其养分含量存在差异, 使得常绿植物分布广泛的热带和高纬度高海拔地区, 植物叶片养分含量较低。
(3)生物地球化学生态位假说(Biogeochemical Niche Hypothesis) (Peñuelas et al., 2008, 2019): 植物需要特定元素组成来维持自身的生长, 不同物种在多种元素含量的高维空间里占据不同的位置和大小, 即化学计量特征的生态位不同。
上述三类假说广泛适用于植物生态化学计量特征的大尺度格局研究中, 有助于理解群落构建和维持机制。
4.1 功能关联假说
4.1.1 生长速率假说
生长速率假说认为生物体的生长速率与C、N、P计量特征密切相关: 随着生长速率的增加, 植物体内的N:P和C:P呈现降低的趋势, 而P含量呈现增加的趋势(Sterner & Elser, 2002; Niklas & Cobb, 2005; 图4A、4B)。该假说的核心基础在于生物体的生长速率的快慢取决于核酸类物质的含量, 而P是核酸类物质的重要组成部分(Sterner & Elser, 2002)。生长速率假说早期在浮游动物、节肢动物和细菌研究中得到了广泛验证(Elser, 2000, 2006; Hessen et al., 2007), 但后来在高等植物中的应用并没有一致的结论。部分研究证明了生长速率假说在高等植物中的适用性(Niklas & Cobb, 2005; Elser et al., 2010), 但也有研究认为生长速率假说的适用性需要考虑养分限制类型(Ågren, 2004, 2008; Yu et al., 2012; Yan et al., 2015)。也就是说, 在N限制的条件下, 生长速率和N:P呈现正相关关系, 不符合生长速率假说; 而在P限制的条件下, 生长速率和N:P呈现负相关关系, 符合生长速率假说(Ågren, 2004, 2008)。这可能是因为在养分限制条件下, N和P在植物组织中存在奢侈吸收的情形, 并不完全被用于生长代谢活动, 使得N:P与生长速率的关系变得模糊。
图4
图4
菟葵和拟南芥的叶片磷含量和氮磷质量比与生长速率的关系。A, B, 改自Niklas和Cobb (2005)。C, D, 数据来自严正兵(2017)拟南芥控制实验数据。
Fig. 4
Bivariate plots of lg-transformed data for growth rates vs leaf phosphorus (P) content and N:P, respectively, of Eranthis hyemalis and Arabidopsis thaliana. A and B are replotted based on Niklas & Cobb (2005). C and D are plotted based on data from Yan’s nutrient enrichment manipulation experiment on A. thaliana (Yan, 2017).
基于模式植物拟南芥的室内施肥实验的结果表明, 生长速率假说在拟南芥中的适用性与养分限制类型有关(Yan et al., 2015)。随着叶片N:P的增加, 拟南芥的生长速率呈现先增加后降低的单峰趋势, 说明当植物受N限制时, 生长速率和N:P呈现正相关关系; 当植物受P限制时, 生长速率和N:P呈现负相关关系(Yan et al., 2015; 图4C、4D)。此外, 也有研究表明, 植物生长速率与蛋白质:RNA含量比值、DNA:RNA含量比值密切相关, 而与N:P的关系较弱(Matzek & Vitousek, 2009; Reef et al., 2010; Loladze & Elser, 2011)。
4.1.2 N:P阈值假说
N:P阈值假说认为存在特定的N:P阈值来判定植物生长的养分限制状况。Koerselman和Meuleman (1996)提出植物N:P < 14时, 植物生长倾向于受到N限制; 植物N:P > 16时, 植物生长倾向于受到P限制; 植物N:P处于14和16之间时, 植物生长可能受到N和P的共同限制, 或不受它们的限制(图5A)。后来, Güsewell (2004)通过综合更多的施肥实验数据, 提出N:P < 10和N:P > 20更适用于判定N和P的养分限制类型。这两种阈值判定标准在提出时, 认为只适用于判定群落水平的养分限制类型, 但后来的研究将该阈值广泛用于判定包含群落和物种水平的植物养分限制类型(Elser et al., 2010; Sardans et al., 2012)。
图5
图5
植物N:P阈值假说的提出和再评估。A, Koerselman和Meuleman (1996)提出N:P的14和16阈值可以作为养分限制类型的判断标准, 改自Koerselman和Meuleman (1996)。B, Yan等(2017)参照Koerselman和Meuleman (1996)的数据分析方法, 并扩充样本量来评估N:P阈值适用性的情形, 改自Yan等(2017)。A和B中养分限制类型是基于野外施肥实验结果判断的, 每个点为单个野外控制实验站点数据。
Fig. 5
Original proposition and re-evaluation of the N:P Threshold Hypothesis. A, The N:P threshold values of 14 and 16 as the judgement standard for N and P limitations proposed by Koerselman & Meuleman (1996), redrawn from Koerselman & Meuleman (1996). B, Re-evaluation of the N:P Threshold Hypothesis by Yan et al. (2017) with a larger dataset following the same statistic method as Koerselman & Meuleman (1996), redrawn from Yan et al. (2017). Nutrient limitation here is judged by results of field nutrient fertilization experiments. Each point indicates a field experiment site.
同时, 越来越多的研究正在质疑N:P阈值在判定养分限制的普适性(Aerts & Chapin III, 1999; Drenovsky & Richards, 2004; Craine et al., 2008)。例如, Han等(2013)认为不同的功能群植物N或P限制的临界N:P值可能不同; 对于常绿针叶林、豆科植物和草本植物, 该临界值分别为10、8-9和12。总体而言, 在植物养分含量充足时, 植物N:P阈值很可能错误地指示植物生长受到养分限制; 当植物养分含量贫瘠时, N:P阈值很可能错误地指示植物生长不受养分限制。例如, 假设植物N和P含量分别为30和4 mg∙g-1, 且该N含量已经高于植物N含量充足的阈值, 而根据N:P阈值假说却得出该植物受到N限制(N:P = 7.5 < 14或10)。同样地, 假定植物N和P含量分别为15和0.60 mg∙g-1, 且该N含量显著低于植物N含量贫瘠的阈值, 而根据N:P阈值假说却得出该植物不受N限制(N:P > 16或20)。通过重新整合野外施肥实验数据以及随机数抽样模拟方法, Yan等(2017)证实了N:P阈值假说在判定养分限制类型时存在失真风险, 且14/16阈值假说的失真风险显著高于10/20阈值假说的失真风险(图5B)。
4.1.3 N-P计量关系的幂指数法则
N和P作为植物体内最重要的两种矿质营养元素, 因其功能上的紧密联系, 它们的含量通常存在幂函数的计量关系(N = βPα, α和β为常数)(Niklas et al., 2005; Reich et al., 2010)。通常情况下, α < 1, 表明P含量的变化速率快于N含量的变化速率。关于α是否恒定是研究该幂指数法则的核心问题。零星的研究结果显示α存在波动, 例如1.0 (McGroddy et al., 2004), 2/3 (Wright et al., 2004; Han et al., 2005), 0.70 (Reich & Oleksyn, 2004)和0.78 (Zhao et al., 2016)。后来, Niklas等发现木本植物和草本植物叶片N-P计量关系的α分别趋于2/3和3/4 (Niklas & Cobb, 2005; Niklas, 2006)。基于中国东部13处森林木本植物的叶片N和P含量数据分析, 发现α存在较大的波动, 其范围为0.57-1.42 (Chen et al., 2013)。尽管如此, 通过整合TRY中叶片N和P含量数据, Reich等(2010)得出α在全球总体程度和不同的功能群间均接近2/3, 由此提出: 植物N和P含量服从相对恒定的幂指数法则; 以往研究得出的α存在变异, 可能是因为样本量不足而导致的。
然而, 最近N-P幂函数关系的大尺度格局分析和控制实验研究表明α存在显著变化。基于更为庞大的全球叶片N和P含量数据量(12 055条记录), Tian等(2018b)对2/3幂指数法则提出了质疑, 并揭示α在不同站点、纬度带、区域和功能群间均存在较大变异(图6A); 该变异可能受植物生长速率、土壤N或P的可利用性、叶片寿命、气候等因素的调控。基于拟南芥的N和P施肥实验也表明N-P幂函数关系受到N和P养分供应状况的调控(Yan et al., 2018)。就同一施肥类型不同施肥水平而言, 随着N (或P)添加水平的升高, 叶片N-P幂函数关系指数明显降低(或上升)(Yan et al., 2018; 图6B、6C); 就不同施肥类型而言, N添加处理下的α显著高于P添加处理(Yan et al., 2018)。这些结果得到了来自西藏高原针对草甸植物长期N和P添加实验结果的验证(Zhang JJ et al., 2018)。
图6
图6
叶片N-P幂函数关系在全球尺度、站点尺度和施肥控制实验的规律。A, 改自Tian等(2018b), 说明叶片N-P幂函数关系在站点尺度存在很大变异。B, C, 改自Yan等(2018), 说明拟南芥叶片N-P幂函数关系受到N或P添加的影响。
Fig. 6
Leaf N-P scaling relationship at global level, site level and nutrient fertilization experimental level. A, Replotted based on Tian et al. (2018b), showing the significant variation of leaf N-P scaling relationship at site levels. B, C, Replotted based on Yan et al. (2018), indicating the effects of N and P fertilizations on leaf N-P scaling relationship of Arabidopsis thaliana.
4.1.4 生产力-养分分配假说和生产力-叶片寿命假说
图7
4.2 环境关联理论和假说
4.2.1 化学计量内稳性理论
图8
图8
化学计量内稳性理论的示意图。A, 生物体中的元素比率随环境中元素比率的变化呈现协同变化, 无内稳性。B, 生物体中的元素比率随环境中元素比率的变化而不变, 呈现出严格的内稳性。图引自Sterner和Elser (2002)。
Fig. 8
Schematic diagram of Stoichiometric Homeostasis Theory. A shows the coordinated variation of organism vs environment nutrient ratios (i.e. no stoichiometric homeostasis). B shows that organism nutrient ratios keep stable with the changing environment nutrient ratios (i.e. strict stoichiometric homeostasis). This figure is replotted from Sterner & Elser (2002).
植物化学计量内稳性的高低受到植物物种、器官、生育时期及植物总体养分含量状况等因素的影响。在物种水平, N和P含量较高、N:P较低的物种内稳性通常较低, 反之内稳性较高(Yu et al., 2011); 内稳性高的物种一般在群落中具有较高的优势度和稳定性, 且内稳性高的生态系统具有较高的生产力和稳定性(Yu et al., 2010, 2015)。在植物器官水平, 幼嫩叶片相比茎、根和衰老叶片, 具有更强的内稳性。这是因为幼嫩叶片的生理功能和养分需求较为重要, 植物需要通过重吸收过程或其他器官的养分转移来保持叶片尽量维持相对恒定的养分组成(Lambers et al., 2008; Schreeg et al., 2014)。同时, 基于拟南芥的施肥实验发现, 同一生育期的繁殖器官相比其他器官具有较强的内稳性(Yan et al., 2016a), 这可能是因为繁殖器官作为代谢活跃的生长中心需要更高的养分含量和更稳定的养分组成, 以促进幼苗生长和植物繁殖(Güsewell, 2004)。在植物的不同生育期, 幼嫩组织和衰老组织的内稳性差异通常与元素的类型有关, 对于在植物组织体内移动性较强的元素(如C、N、P、K), 幼嫩组织具有较强的内稳性(Han et al., 2013; Schreeg et al., 2014; Yan et al., 2016a)。在不同的生长发育阶段, 不同元素的内稳性可能反映了相应阶段的营养元素限制情况, 如在苋(Amaranthus mangostanus)的苗期, N的内稳性高于P, 而开花期则相反, 表明该植物在营养生长阶段更容易受N限制, 而在繁殖生长阶段则相对受P的限制(Peng et al., 2016); 对于在植物组织体内移动性较弱的元素(如Ca), 衰老组织具有较强的内稳性(Güsewell, 2004; Yan et al., 2018)。上述结果说明营养器官和衰老组织的养分组成对移动性较强的营养元素在环境中的变异具有较强的指示作用, 而对移动性较弱的营养元素具有较弱的环境指示作用(Vergutz et al., 2012; Schreeg et al., 2014; Yan et al., 2016a, 2018)。
4.2.2 限制元素稳定性假说
限制元素稳定性假说认为, 由于生理和养分平衡的制约, 限制元素在植物体内的含量具有相对稳定性, 其对环境变化的响应较为稳定(Han et al., 2011)。通过整合分析中国植物11种矿质元素(N、P、K、Ca、Mg、S、Fe、Mn、Al、Si、Na)含量的变异, Han等(2011)提出了该假说, 发现那些植物需求高但经常受限制的营养元素, 通常具有较低的变异系数和较高的稳定性(图9)。土壤肥力和全球化肥使用量等数据印证了该假说。这也符合化学计量内稳性理论: 植物为了维持自身功能的平衡, 对于自身的养分组成做出选择性的调整, 尽量减少重要且相对受限的元素含量的波动, 维持重要器官的养分组成相对稳定(Schreeg et al., 2014; Yan et al., 2016a)。该假说系统地解释了大尺度环境梯度中植物所受的基本生态化学计量(养分平衡)的限制, 为植物营养生态学研究提供了一种新方法。
图9
限制元素稳定性假说已经在物种、种群和群落尺度都得到了广泛验证。基于拟南芥的施肥实验, Yan等(2018)发现该假说在拟南芥中也同样适用, 叶片C、N、P、K、Ca和Mg含量与它们的变异系数呈负相关关系。对巴西季雨林叶片化学元素计量特征进行研究发现, N和P含量相比其他元素(Mn、Cu、Zn、Fe、B等)含量在不同土壤条件、物种和植被类型中的变异较小, 符合该假说(Miatto & Batalha, 2016)。针对中国主要森林类型叶片和根系16种元素计量关系的研究发现, 叶片和根系中含量较高的元素变异较低, 证实了限制元素稳定性假说, 并发现多种元素的变异性主要受到植物类群, 而不是外界环境条件的影响(Zhao et al., 2016)。
4.2.3 叶片养分含量稳定假说
图10
图10
中国不同生物群区植物器官间氮(N)磷(P)含量的关系图。叶片氮磷含量相比茎和根氮磷含量的斜率均小于1, 说明叶片养分含量相比根、茎养分含量更稳定, 对外界环境条件的响应程度更低。该结果支持“叶片养分含量稳定假说”。改自Tang等(2018)。
Fig. 10
Scaling relationships of nitrogen (N) and phosphorus (P) content among different organs from different biomes in China. The scaling exponents from leaf vs stem and leaf nutrient vs root nutrient relationships are all below 1, suggesting that nutrient content in leaves relative to stems and roots is more stable and less sensitive to environmental changes. These results support the Stable Leaf Nutrient Content Hypothesis. This figure is replotted based on Tang et al. (2018).
基于中国4 149个样地常见物种不同器官养分含量和生物量的野外调查取样, Tang等(2018)将不同器官(根、茎、叶)间的C、N、P含量通过生物量加权的方法, 研究了群落水平不同器官间养分含量的幂函数计量关系, 提出了该假说。个体和单个尺度上的内稳性研究也发现, 叶片作为植物生长较活跃的组织, 其生长代谢功能依赖于较稳定的养分组成, 因此在响应外界养分环境变化时, 相比根和茎而言表现出较高的内稳性(Schreeg et al., 2014; Yan et al., 2016a)。这些研究表明叶片养分含量假说在个体、物种和群落水平均得到了验证, 指示根和茎化学计量特征能更加准确地反映植物养分组成对外界环境的响应, 具有更高的可塑性和敏感性, 而以往研究通过测定叶片养分含量来表征植物的养分限制可能存在较大的不确定性(Yan et al., 2017)。需要注意的是, 虽然叶片养分含量稳定假说在分析中国所有样地水平的数据时是成立的, 但在分析局域尺度的小枝和叶片养分含量的幂函数关系时发现, 该关系的幂指数存在明显的纬度梯度: 中低纬度地区幂指数大于1, 说明小枝比叶片养分含量变化更为敏感, 而高纬度地区幂指数小于1, 说明叶片养分含量变化更为敏感(Yan et al., 2016b)。因而, 大尺度的叶片养分含量稳定假说在站点和局域尺度的适用性仍需要进一步检验。
4.2.4 温度-生物地球化学假说和温度-植物生理假说
关于温度对植物生态化学计量特征的影响, 目前有两个对应的假说: (1)温度-生物地球化学假说, 认为温度影响着土壤微生物的活性, 从而影响着土壤N和P的矿化速率, 因此在低温条件下, 土壤N和P的可利用性较低, 导致植物叶片N和P含量偏低(Aerts & Chapin III, 1999; Reich & Oleksyn, 2004); (2)温度-植物生理假说, 认为植物的生长代谢速率受温度的调控, 在低温条件下, 植物的生理过程受到抑制, 为了补偿植物较低的生长代谢速率过程, 植物可能会启动相应的温度敏感调控机制, 来提高植物的N和P含量, 弥补酶效率和RNA合成效率降低的影响, 这也解释了高寒和高纬度生境的植物叶片具有较高N和P含量的现象(Weih & Karlsson, 2001; Reich & Oleksyn, 2004)。
对全球陆生植物N和P含量随温度的变化进行研究发现, 随着温度的增加, 叶片N和P含量呈现先增加后降低的趋势, 且拐点大概在5 ℃左右, 推测5 ℃以下主要是温度-生物地球化学假说占主导, 5 ℃以上主要是温度-植物生理假说占主导(Reich & Oleksyn, 2004)。如果将青藏高原的植物叶片N含量数据加入Reich和Oleksyn (2004)的数据库中, 在5 ℃以下, 叶片N含量随年平均气温升高而升高的趋势消失了, 间接否定了温度-生物地球化学假说(He et al., 2006a)。他们认为, Reich和Oleksyn (2004)观察到的低温趋势可能是由于5 ℃以下的数据太少, 或搜集来的数据的采样方法和标准不统一所造成的。对中国北方温带灌丛C、N和P化学计量特征的研究表明, 随着年平均气温的增加, 植物P含量呈降低趋势, 可能与P含量用来补偿低温导致的代谢速率降低有关, 符合温度-植物生理假说(Yang et al., 2016)。
4.2.5 土壤基质年龄假说
土壤基质年龄假说认为土壤N和P含量在较年轻和老龄土壤中较低, 在年轻土壤中含量较高, 因此, 土壤年龄较老的热带地区, 淋溶较为严重, 肥力较低, 植物N和P含量在低纬度地区较低(Reich & Oleksyn, 2004)。此外, 高纬度地区的土壤较为年轻, 从母岩中释放P的能力较强, 而母岩中N含量较低; 热带地区土壤较为古老, 表面母质淋溶和风化较为严重, P从母岩中被释放出来的能力较弱, 土壤中P含量较为缺乏(Walker & Syers, 1976; Vitousek et al., 2010)。因此, 该假说认为, 高纬度地区土壤较为年轻, N:P较低, 通常为N限制; 而低纬度地区的土壤发育较为古老, N:P较高, 通常为P限制(Reich & Oleksyn, 2004; Elser et al., 2007; Sardans et al., 2012)。
4.2.6 相对重吸收假说
基于全球植物叶片N和P养分重吸收数据的整合分析, Han等(2013)提出了相对重吸收假说, 认为N重吸收效率(NRE)和P重吸收效率(PRE)的差值与成熟叶片的N:P存在负相关关系, 且存在NRE = PRE的N:P临界值; 当N:P小于该临界值, NRE > PRE, 指示植物可能受到N限制; 当N:P大于该临界值, NRE < PRE, 指示植物可能受到P限制(图11)。分析该数据库中的所有物种发现, N:P的临界值大约为15; 尽管常绿落叶、落叶阔叶、针叶和固氮植物在NRE = PRE时的N:P临界值不尽相同, 但都较好地符合了相对重吸收假说的规律(Han et al., 2013)。针对苋和拟南芥的养分添加实验也都证明了相对重吸收假说的合理性, 但N:P临界值在这两种物种间存在差异(Yan et al., 2015; Peng et al., 2016)。
图11
图11
相对重吸收假说提出的数据分析图, 改自Han等(2013)。纵坐标为氮重吸收效率(NRE)与磷重吸收效率(PRE)的差值。①沙漠; ②热带雨林; ③亚热带森林; ④湿地; ⑤中国温带森林; ⑥温带森林; ⑦中国北方森林; ⑧草甸; ⑨北方森林; ⑩欧石南灌丛; ⑪高草草原。
Fig. 11
Diagram of Relative Resorption Hypothesis, replotted based on Han et al. (2013). The y-axis shows the relative resorption efficiency of nitrogen (N) and phosphorus (P) (NRE - PRE). ① desert; ② tropical forest; ③ subtropical forest; ④ wetland; ⑤ China temperate forest; ⑥ temperate forest; ⑦ China boreal forest; ⑧ meadow; ⑨ boreal forest; ⑩ heathland; ⑪ tallgrass prairie.
类似地, NRE:PRE也可以用来表征相对重吸收效率。Reed等(2012)提出NRE:PRE随着纬度的升高而增加, 随着年平均气温升高和年降水量增加而降低, 预示着高纬度地区的植物倾向于N限制, 而低纬度地区的植物倾向于P限制。最近一项研究基于相对重吸收的思想, 用优势树种的NRE:PRE探究了生态系统尺度的养分限制(Du et al., 2020)。当优势树种的NRE:PRE > 1 (或ln (NRE:PRE) > 0)时, 指示生态系统处于N限制, 反之处于P限制; 基于NRE:PRE判定的养分限制类型和施肥实验得到的结论是一致的(Du et al., 2020)。同时, 基于NRE:PRE的分析发现全球18%的自然生态系统受到N的限制, 43%的地区受到P的限制, 剩下39%受到N和P的共同限制或微弱限制。
4.3 进化关联假说
4.3.1 物种组成假说和常绿-落叶假说
物种组成假说认为物种或生活型组成差异影响植物叶片化学计量特征的生物地理格局(Reich & Oleksyn, 2004)。如果特定地区的优势物种或生活型本身具有较高的叶片养分含量, 那么该地区的植物叶片养分含量较高, 反之亦然。对中国草地植物C、N和P化学计量特征变异及其驱动因素的研究发现, 物种组成是最主要的驱动因素, 温度主要通过改变物种组成而间接影响化学计量特征的变异, 即支持物种组成假说(He et al., 2006a)。分析中国陆生植物11种营养元素含量的生物地理格局, 发现这些元素含量的经纬度格局主要是由气候、土壤和植物功能群类型驱动的, 其中植物功能群类型起着最主要的作用, 间接证明了物种组成假说的合理性(Han et al., 2011)。类似地, 研究中国主要森林群区植物叶片和根系16种营养元素含量的空间变异, 发现大部分变异主要归因于植物类群, 符合物种组成假说; 同时, 相比微量元素而言, 大量元素含量的变化范围较窄, 更容易受到植物类群的调控(Zhao et al., 2016)。
在物种组成假说基础上, Reich和Oleksyn (2004)提出了常绿-落叶假说。该假说认为, 常绿植物的叶片寿命通常比落叶植物长, 而叶片寿命和叶片养分含量密切相关, 寿命较长的叶片通常含有较低的养分含量, 因此, 在常绿植物分布广泛的热带和高纬度高海拔地区, 植物叶片养分含量较低。常绿-落叶植物的组成比例不同进而驱动形成叶片N和P含量的生物地理格局, 常绿植物通常分布在热带地区和
4.3.2 生物地球化学生态位假说
生物体需要特定数量和比例的营养元素来维持自身的生长, 不同物种因其功能和生活策略的差异, 具有不同的生化结构和特定的生理代谢过程, 因而具有不同的营养元素需求, 在多种元素含量的n维空间里占据不同的位置和大小, 即生物地球化学生态位假说(图12; Peñuelas et al., 2008, 2019)。该假说的成立基于以下认知: (1)不同的物种应该具有不同的生物地球化学生态位, 并且这种生态位差异随着谱系距离和进化时间的增加而扩大; (2)同一地点共存的物种应该具有差异较大的生物地球化学生态位来减小竞争的压力; (3)生物地球化学生态位会随着环境的波动而适当改变, 以满足物种适应新环境的竞争压力(Peñuelas et al., 2019)。因此, 生物地球化学生态位的特征受到功能群、谱系距离、竞争强度和内稳性大小等综合因素的影响, 这些因素对生态系统功能具有一定的反馈作用, 最终成为生命和环境演化的驱动力。
图12
图12
生物地球化学生态位假说示意图, 改自Peñuelas等(2019)。A, 共生植物在多种元素化学计量特征的双主成分上所呈现的生物地球化学生态位。B, 干旱实验处理下共生植物的生物地球化学生态位的偏移。图中不同颜色组成的单个椭圆形表示某个特定的生物地球化学生态位。
Fig. 12
Diagram of Biogeochemical Niche Hypothesis, modified from Peñuelas et al. (2019). A shows biogeochemical niche segregation of multi-nutrients among coexisting plant species based on the principal component analysis. B shows the shift in biogeochemical niche among coexisting plant species in a drought experiment. Ellipses in different colours show specific biogeochemical niches.
已有研究已经证实了生物地球化学生态位假说的适用性(Sardans et al., 2021)。例如, 对西班牙加泰罗尼亚地区森林植物元素组成和计量特征进行研究, 发现气候条件和分类群差异导致地中海炎热干旱地区的物种相比非地中海地区的物种具有较低的叶片N、P和K含量, 并表明生物地球化学生态位是物种长期适应生物和非生物因子的结果(Sardans & Peñuelas, 2014)。分析入侵物种和本地物种的生物地球化学生态位差异, 发现入侵物种对大量和微量元素具有更强的获取能力, 因而解释了外来物种成功入侵的原因(Peñuelas et al., 2010)。探究不同群落组成(单作和混作)条件下的矮灌木和禾草的多元素特征, 发现不同的植物群落组成会影响物种的生物地球化学生态位(Urbina et al., 2017)。对中国森林根系元素组成的分析也进一步证实了生物地球化学生态位假说: 群落水平的根系化学元素组成沿着环境梯度呈现系统性的变化, 受到大尺度环境条件的过滤, 尤其是土壤和气候因子的影响(Zhao et al., 2018)。对中国东北地区樟子松(Pinus sylvestris var. mongolica)沿环境梯度变化的化学计量特征进行研究, 发现同一物种的化学计量特征变异随着干旱程度的增加、土壤养分可利用性的减少而降低, 说明樟子松的生物地球化学生态位随着环境胁迫的增加而改变(He P et al., 2019)。尽管如此, 生物地球化学生态位假说如何与群落结构、生物多样性以及生态系统功能相联系仍需在今后开展深入研究。
5 结论与展望
近30年来, 植物生态化学计量学已成为生态学研究的热点之一, 尤其在中国得到了迅速发展。由于篇幅所限, 本文在介绍植物生态化学计量发展简史的基础上, 仅重点综述了当前化学计量学在以下3个方面的研究进展: (1)植物主要器官的化学计量特征及其驱动因素; (2)施肥对植物生态化学计量特征的影响; (3)植物生态化学计量的主要假说和理论。可以概括总结为如下结论: (1)植物生态化学计量学研究经历了思想萌芽期、假说奠基期和理论构建期3个发展阶段; (2)植物主要器官的化学计量特征呈现出显著的生物地理格局, 这种格局是气候、土壤和植物功能群类型共同作用的结果; (3)养分变化显著改变了植物生态化学计量特征的平衡状态; (4)为解释植物生态化学计量特征及其变化的机制问题, 一些学者相继提出与植物生长功能、环境因子和植物进化历史相关联的多种理论或假说。
尽管国内外已有大量关于植物生态化学计量特征及其驱动因素的研究, 但仍然有一些重要的科学问题和方向需要进一步去探究。为加强植物生态化学计量特征及其相关内容的研究, 我们提出以下10个未来应该重点关注的方面。
(1)就元素类型而言, 当前植物生态化学计量学研究主要聚焦于C、N、P等元素, 对于其他重要的营养元素(如K、Ca、Mg、Si、Fe、Na、Zn等)的化学计量特征的研究还十分缺乏。虽然已有少量研究报道了它们的大尺度格局, 但关于它们变异的形成和驱动机制尚不明确。
(2)就器官类型而言, 当前植物生态化学计量学研究仍聚焦在叶片, 对于根系化学计量特征的研究虽陆续有较多报道, 但对于茎和繁殖器官化学计量特征的规律知之甚少; 并且, 将植物视为一个整体, 不同器官间化学计量之间的关联如何更缺乏认知。回答这些问题将有助于理解植物的养分分配和利用策略, 进一步了解植物对环境的响应和适应机制。
(3)从生态系统地上-地下关系的角度分析, 土壤微生物-土壤养分-植物生态化学计量特征间的耦合关系是植物生态学和全球变化生态学中的一个热点问题, 但不同土壤微生物在土壤-植物互作中发挥的功能尚不清晰。借助高通量测序等技术, 探明土壤微生物关键种和群落的化学计量特征及其功能, 对于理解土壤-植物生态化学计量特征变化规律和陆地生态系统C、N、P循环过程具有重要意义。
(4)光照条件如何影响植物叶片化学计量特征的大尺度格局尚不清楚。以往研究多采集生长季节的向阳叶片, 分析其化学计量特征。事实上, 对于高大乔木, 我们很难采集到树冠顶层的叶片, 只有依赖森林塔吊装置方能实现这一目标。这意味着, 许多研究只能采集到冠层中部或下部的叶片, 其生长状况受到了遮阴的影响。因而, 今后研究需要更多地结合塔吊等装置, 通过采集林冠间和林冠内部垂直结构的样品来分析光照条件对化学计量特征的影响。
(5)季节变化和叶片年龄如何塑造植物生态化学计量特征。以往研究多采集生长季节的成熟叶片来分析其化学计量特征, 而对于叶片化学计量特征的季节动态研究甚少, 同时叶片的发育阶段, 即叶片年龄对化学计量特征的影响在不同物种间的差异亦不清楚。探究这些问题, 有助于我们在更精细的时间尺度上分析植物的养分利用和限制状况, 为回答植物动态生产力提供相关机制解释。
(6)关于个体-种群-群落-生态系统间化学计量特征的尺度推移的研究十分缺乏。从群落构建和维持、生态系统功能和动态等角度, 我们需要分析更大空间尺度的植物生态化学计量特征规律以帮助了解植物养分循环过程对大尺度生态系统功能的调控作用。然而, 当前绝大多数研究只停留在叶片尺度上, 大多只是通过简单的生物量加权方法进行群落尺度的分析。今后, 如何结合新型遥感技术手段和稳健的过程模型来帮助回答该问题是一个重要的研究方向。
(7)植物生态化学计量研究应该从描述性向机制性研究转变。当前许多化学计量研究仍是聚焦在不同器官化学计量特征及其影响因素方面。植物生态化学计量特征的本质是回答植物的养分吸收、利用和循环过程以及植物的养分限制状况, 这与许多生态系统功能密切相关。今后的研究应聚焦到化学计量特征与生态系统过程和功能的关联, 回答养分状况对后者的调控作用。
(8)在全球变化背景下, 仍需加强未来植物生态化学计量特征如何响应并调控陆地生态系统碳循环过程的研究。许多全球变化因子(如温度升高、CO2浓度上升、氮沉降等)均会改变植物生态化学计量特征, 影响植物的养分平衡状态, 进而调节植物光合作用驱动陆地碳汇能力的动态变化。因此, 在今后的地球系统模型中, 如何定量刻画化学计量特征的动态变化对碳吸收利用过程的调控作用, 需从机制厘清和模型优化的角度深入研究。
(9)化学计量在植物-动物间的耦合关系以及随食物网的级联效应中的应用属于前沿方向, 研究尚处于起步阶段。研究该问题有助于理解养分元素在不同营养级间的循环过程, 为从植物生态化学计量角度评估和预测更高营养级的养分吸收、利用和限制状况提供了新思路和新视角, 有利于促进植物生态化学计量学在指导动植物生产中的应用, 例如, “植物工厂”中的无土栽培, “草牧业”中牧草培育和牲畜的科学喂养等。
(10)验证和拓展植物生态化学计量学的现有假说和理论对于解释一系列生态学现象和过程具有重要意义。今后, 除关注植物生态化学计量特征的实际应用研究外, 还应加强其理论研究, 促进植物生态化学计量学与生命科学(如生物化学、细胞生物学等)和地理学的交叉与融合。
参考文献
Plant and soil responses to chronic nitrogen additions at the Harvard Forest, Massachusetts
DOI:10.2307/1941798 URL [本文引用: 1]
Above-ground nutrient turnover and net primary production of an evergreen and a deciduous species in a heathland ecosystem
DOI:10.2307/2260754 URL [本文引用: 1]
The mineral nutrition of wild plants revisited: a re-evaluation of processes and patterns
Ideal nutrient productivities and nutrient proportions in plant growth
The C:N:P stoichiometry of autotrophs- Theory and observations
DOI:10.1111/ele.2004.7.issue-3 URL [本文引用: 3]
Stoichiometry and nutrition of plant growth in natural communities
DOI:10.1146/ecolsys.2008.39.issue-1 URL [本文引用: 3]
Plant stoichiometry at different scales: element concentration patterns reflect environment more than genotype
DOI:10.1111/nph.2012.194.issue-4 URL [本文引用: 1]
Leaf analysis and shoot production in Phragmites
DOI:10.2307/3564972 URL [本文引用: 1]
Effects of irrigation and fertilization on mineral nutrients in Scots pine needles
Variation in the elemental content of Eichhornia crassipes
DOI:10.1007/BF00036546 URL [本文引用: 1]
Experimental investigations into the mineral nutrition of several grass species: Part III. Phosphate level
DOI:10.2307/2257338 URL [本文引用: 1]
Distribution patterns and driving factors of leaf C, N and P stoichiometry of coniferous species on the eastern Qinghai-Xizang Plateau, China
DOI:10.17521/cjpe.2019.0221
[本文引用: 1]
<p id="C2"><b><i>Aims</i></b> The leaf stoichiometry and potential driving factors play a vital role in understanding the distribution patterns of plant community and predicting the plant responses to environmental changes. In this study, we aimed to investigate the spatial distribution patterns and driving factors of leaf carbon (C), nitrogen (N) and phosphorus (P) stoichiometry of coniferous species on the eastern Qinghai-Xizang Plateau, China. <br><b><i>Methods</i></b> We collected leaf and soil samples from 29 coniferous tree species at 84 sampling sites on the eastern Qinghai-Xizang Plateau. Linear fitting was used to analyze the variation patterns of leaf stoichiometry along geographical and climatic gradients. Partial redundancy analysis was used to characterize the relative contributions of climate and soil factors to leaf stoichiometry variation patterns. <br><b><i>Important findings</i></b> (1) At the level of family and genus, C and N concentrations as well as C:N of leaves were significantly different across distinct conifer species. The leaf N:P was less than 14, indicating that conifer species in the study region were mainly N-limited. (2) Leaf N and P concentrations showed a consistent distribution pattern along environmental gradients. Specifically, N and P concentrations of leaves were significantly decreased with elevated latitude and altitude, while remarkably increased with the increase of mean annual temperature (MAT) and mean annual precipitation (MAP). In comparison, leaf C concentration had no significant correlation with latitude, altitude, MAT or MAP. (3) The leaf C:N and C:P showed an opposite distribution pattern with leaf N and P concentrations, which significantly increased with elevated latitude and altitude, while markedly declined with the increase of MAT and MAP. Leaf N:P had no significant correlation with altitude, MAT or MAP. (4) The main driving factors of leaf C, N, P concentrations and their stoichiometric characteristics were different. Specifically, soil properties were the main driving factors accounting for the variations of leaf C concentration and N:P. The variations of leaf N and P concentrations as well as ratios of C:N and C:P were primarily explained by climatic factors. Collectively, variations of leaf stoichiometry of coniferous species along environmental gradients in the study region provided a compelling support for the Temperature Biogeochemistry Hypothesis. These findings largely improved the understanding of the distribution patterns and driving mechanism of leaf stoichiometry under changing environments.</p>
青藏高原东缘主要针叶树种叶片碳氮磷化学计量分布格局及其驱动因素
DOI:10.17521/cjpe.2019.0221
[本文引用: 1]
理解植物叶片化学计量特征及其驱动因素对认识植物种群分布规律及预测植物对环境变化响应具有重要意义。该研究采集了青藏高原东缘针叶林84个样点共29种主要针叶树种叶片, 探讨该区域常绿针叶树种叶片碳(C)、氮(N)、磷(P)化学计量特征和分布格局及其驱动因素。结果表明: (1)在科和属水平上, 不同针叶树种叶片C、N含量和C:N差异显著; 叶片N:P < 14, 表明该区域针叶树种主要受N限制。(2)叶片N、P含量在环境梯度上表现出一致的分布规律: 均呈现出随纬度和海拔增加而显著降低, 随年平均气温(MAT)和年降水量(MAP)增加而显著增加的趋势; 而叶片C含量与纬度、海拔、MAT和MAP均未表现出显著相关性。(3)叶片C:N、C:P呈现出与N、P含量变化相反的分布格局: 均随纬度和海拔增加而显著增加, 随MAT和MAP增加而显著降低; 而叶片N:P与海拔、MAT和MAP均无显著相关性。(4)进一步分析表明, 叶片C、N、P含量及其化学计量比的主要驱动因素不尽相同。具体而言: 土壤特性是叶片C含量和N:P变异的主要驱动因子, 而叶片N、P含量和C:N、C:P的变异主要由气候因素决定。总之, 该区域针叶树种叶片化学计量沿环境梯度的变异规律有力地支持了温度生物地球化学假说, 在一定程度上丰富了对环境变化下植物叶片化学计量分布格局及其驱动机制的认识。
Global biodiversity, stoichiometry and ecosystem function responses to human-induced C-N-P imbalances
DOI:10.1016/j.jplph.2014.07.022
PMID:25270104
[本文引用: 1]
Global change analyses usually consider biodiversity as a global asset that needs to be preserved. Biodiversity is frequently analysed mainly as a response variable affected by diverse environmental drivers. However, recent studies highlight that gradients of biodiversity are associated with gradual changes in the distribution of key dominant functional groups characterized by distinctive traits and stoichiometry, which in turn often define the rates of ecosystem processes and nutrient cycling. Moreover, pervasive links have been reported between biodiversity, food web structure, ecosystem function and species stoichiometry. Here we review current global stoichiometric gradients and how future distributional shifts in key functional groups may in turn influence basic ecosystem functions (production, nutrient cycling, decomposition) and therefore could exert a feedback effect on stoichiometric gradients. The C-N-P stoichiometry of most primary producers (phytoplankton, algae, plants) has been linked to functional trait continua (i.e. to major axes of phenotypic variation observed in inter-specific analyses of multiple traits). In contrast, the C-N-P stoichiometry of higher-level consumers remains less precisely quantified in many taxonomic groups. We show that significant links are observed between trait continua across trophic levels. In spite of recent advances, the future reciprocal feedbacks between key functional groups, biodiversity and ecosystem functions remain largely uncertain. The reported evidence, however, highlights the key role of stoichiometric traits and suggests the need of a progressive shift towards an ecosystemic and stoichiometric perspective in global biodiversity analyses. Copyright © 2014 Elsevier GmbH. All rights reserved.
Nutrient allocation and responses to defoliation in tundra plants
DOI:10.2307/1550500 URL [本文引用: 3]
The mineral nutrition of wild plants
DOI:10.1146/ecolsys.1980.11.issue-1 URL [本文引用: 2]
Seasonal changes in nitrogen and phosphorus fractions and autumn retranslocation in evergreen and deciduous taiga trees
DOI:10.2307/1937083 URL [本文引用: 1]
Soil temperature and nutrient cycling in the tussock growth form of Eriophorum vaginatum
DOI:10.2307/2259343 URL [本文引用: 1]
Towards a worldwide wood economics spectrum
DOI:10.1111/ele.2009.12.issue-4 URL [本文引用: 1]
Nitrogen saturation in humid tropical forests after 6 years of nitrogen and phosphorus addition: hypothesis testing
DOI:10.1111/fec.2016.30.issue-2 URL [本文引用: 1]
Leaf nitrogen and phosphorus concentrations of woody plants differ in responses to climate, soil and plant growth form
DOI:10.1111/more.2013.36.issue-2 URL [本文引用: 2]
The research advances and prospect of ecological stoichiometry
生态化学计量学研究进展
On the ratio of nitrogen to phosphorus in the sea
DOI:10.1017/S0025315400011930 URL [本文引用: 1]
Nutrient concentration ratios and co-limitation in South African grasslands
DOI:10.1111/nph.2008.179.issue-3 URL [本文引用: 1]
A latitudinal gradient in seed nutrients of the forest herb Anemone nemorosa
DOI:10.1111/j.1438-8677.2010.00404.x
PMID:21489100
[本文引用: 1]
The nutrient concentration in seeds determines many aspects of potential success of the sexual reproductive phase of plants, including the seed predation probability, efficiency of seed dispersal and seedling performance. Despite considerable research interest in latitudinal gradients of foliar nutrients, a similar gradient for seeds remains unexplored. We investigated a potential latitudinal gradient in seed nutrient concentrations within the widespread European understorey forest herb Anemone nemorosa L. We sampled seeds of A. nemorosa in 15 populations along a 1900-km long latitudinal gradient at three to seven seed collection dates post-anthesis and investigated the relative effects of growing degree-hours >5 °C, soil characteristics and latitude on seed nutrient concentrations. Seed nitrogen, nitrogen:phosphorus ratio and calcium concentration decreased towards northern latitudes, while carbon:nitrogen ratios increased. When taking differences in growing degree-hours and measured soil characteristics into account and only considering the most mature seeds, the latitudinal decline remained particularly significant for seed nitrogen concentration. We argue that the decline in seed nitrogen concentration can be attributed to northward decreasing seed provisioning due to lower soil nitrogen availability or greater investment in clonal reproduction. This pattern may have large implications for the reproductive performance of this forest herb as the degree of seed provisioning ultimately co-determines seedling survival and reproductive success.© 2010 German Botanical Society and The Royal Botanical Society of the Netherlands.
Responses of terrestrial ecosystem phosphorus cycling to nitrogen addition: a meta-analysis
DOI:10.1111/geb.2017.26.issue-6 URL [本文引用: 1]
Critical N:P values: predicting nutrient deficiencies in desert shrublands
DOI:10.1023/B:PLSO.0000020945.09809.3d URL [本文引用: 1]
Global patterns of terrestrial nitrogen and phosphorus limitation
DOI:10.1038/s41561-019-0530-4 URL [本文引用: 3]
NEECF: a project of nutrient enrichment experiments in China’s forests
DOI:10.1093/jpe/rtt008 URL [本文引用: 3]
Leaf life span and nutrient resorption as determinants of plant nutrient conservation in temperate-arctic regions
DOI:10.1046/j.1469-8137.1999.00429.x URL [本文引用: 1]
Biological stoichiometry: a chemical bridge between ecosystem ecology and evolutionary biology
Ecological stoichiometry: from sea to lake to land
DOI:10.1016/S0169-5347(00)01956-X URL [本文引用: 2]
Nutritional constraints in terrestrial and freshwater food webs
DOI:10.1038/35046058 URL [本文引用: 1]
Global analysis of nitrogen and phosphorus limitation of primary producers in freshwater, marine and terrestrial ecosystems
DOI:10.1111/ele.2007.10.issue-12 URL [本文引用: 3]
Organism size, life history, and N:P stoichiometry: toward a unified view of cellular and ecosystem processes
DOI:10.2307/1312897 URL [本文引用: 2]
Biological stoichiometry of plant production: metabolism, scaling and ecological response to global change
DOI:10.1111/j.1469-8137.2010.03214.x
PMID:20298486
[本文引用: 3]
Biological stoichiometry theory considers the balance of multiple chemical elements in living systems, whereas metabolic scaling theory considers how size affects metabolic properties from cells to ecosystems. We review recent developments integrating biological stoichiometry and metabolic scaling theories in the context of plant ecology and global change. Although vascular plants exhibit wide variation in foliar carbon:nitrogen:phosphorus ratios, they exhibit a higher degree of 'stoichiometric homeostasis' than previously appreciated. Thus, terrestrial carbon:nitrogen:phosphorus stoichiometry will reflect the effects of adjustment to local growth conditions as well as species' replacements. Plant stoichiometry exhibits size scaling, as foliar nutrient concentration decreases with increasing plant size, especially for phosphorus. Thus, small plants have lower nitrogen:phosphorus ratios. Furthermore, foliar nutrient concentration is reflected in other tissues (root, reproductive, support), permitting the development of empirical models of production that scale from tissue to whole-plant levels. Plant stoichiometry exhibits large-scale macroecological patterns, including stronger latitudinal trends and environmental correlations for phosphorus concentration (relative to nitrogen) and a positive correlation between nutrient concentrations and geographic range size. Given this emerging knowledge of how plant nutrients respond to environmental variables and are connected to size, the effects of global change factors (such as carbon dioxide, temperature, nitrogen deposition) can be better understood.
Phosphorus and nitrogen limitation of phytoplankton growth in the freshwaters of North America: a review and critique of experimental enrichments
DOI:10.1139/f90-165 URL [本文引用: 1]
Photosynthesis and nitrogen relationships in leaves of C3 plants
DOI:10.1007/BF00377192
PMID:28311896
[本文引用: 1]
The photosynthetic capacity of leaves is related to the nitrogen content primarily bacause the proteins of the Calvin cycle and thylakoids represent the majority of leaf nitrogen. To a first approximation, thylakoid nitrogen is proportional to the chlorophyll content (50 mol thylakoid N mol Chl). Within species there are strong linear relationships between nitrogen and both RuBP carboxylase and chlorophyll. With increasing nitrogen per unit leaf area, the proportion of total leaf nitrogen in the thylakoids remains the same while the proportion in soluble protein increases. In many species, growth under lower irradiance greatly increases the partitioning of nitrogen into chlorophyll and the thylakoids, while the electron transport capacity per unit of chlorophyll declines. If growth irradiance influences the relationship between photosynthetic capacity and nitrogen content, predicting nitrogen distribution between leaves in a canopy becomes more complicated. When both photosynthetic capacity and leaf nitrogen content are expressed on the basis of leaf area, considerable variation in the photosynthetic capacity for a given leaf nitrogen content is found between species. The variation reflects different strategies of nitrogen partitioning, the electron transport capacity per unit of chlorophyll and the specific activity of RuBP carboxylase. Survival in certain environments clearly does not require maximising photosynthetic capacity for a given leaf nitrogen content. Species that flourish in the shade partition relatively more nitrogen into the thylakoids, although this is associated with lower photosynthetic capacity per unit of nitrogen.
Plant root N and P levels and their relationship to geographical and climate factors in a Chinese grassland transect
中国草地样带植物根系N、P元素特征及其与地理气候因子的关系
Chemical element background and its distribution in arctic soils
北极冻土的化学元素背景及其分布特征
Contents and distribution of chemical elements in arctic ice.
北极冰化学元素含量及其分布
Developing an indicator of nutrient enrichment in coastal estuaries and lagoons using tissue nitrogen content of the opportunistic alga, Enteromorpha intestinalis (L. Link)
DOI:10.1016/S0022-0981(98)00085-9 URL [本文引用: 2]
Nitrogen cycles: past, present, and future
DOI:10.1007/s10533-004-0370-0 URL [本文引用: 1]
Linking above- and belowground traits to soil and climate variables: an integrated database on China’s grassland species
DOI:10.1002/ecy.1780
PMID:28241374
[本文引用: 1]
Knowledge of plant functional traits and trait-environment interactions is important for characterizing species strategies and understanding ecological processes. However, comprehensive field data on both above- and belowground traits, together with their environmental variables are scarce. Biome-scale studies are particularly lacking. Here we present two large-scale data sets that include functional traits of leaves and fine roots and their corresponding soil and climatic variables in China's grasslands. Leaf, fine root, and soil samples were collected in three biogeographic regions: temperate grassland on the Inner Mongolia Plateau, alpine grassland on the Tibetan Plateau, and mountain grassland in the Xinjiang mountain areas. Field data were collected over two periods. The first data set collected between 2003 and 2004 includes 13 foliar traits (leaf mass per area, LMA; photosynthetic nitrogen use efficiency, PNUE; water use efficiency, WUE; stomatal conductance for water vapor, Gs; transpiration rate, TR; mass- and area-based photosynthetic capacity, Amass and Aarea; mass- and area-based carbon concentrations, Cmass and Carea; nitrogen concentrations, Nmass and Narea; and phosphorus concentrations, Pmass and Parea) for 170 species at 173 sites. The second data set collected between 2006 and 2007 includes six sets of analogous traits for both leaves and fine roots (C, N, and P concentrations; leaf thickness/root diameter; specific leaf area, SLA; specific root length, SRL; and tissue density) for 139 species at 82 sites, along with soil attributes (soil total and organic carbon, STC and SOC; total and available N, STN and SAN; total and available P, STP and SAP; pH, bulk density, and moisture). Moreover, associated information was also gathered, including geographical location (latitude, longitude, and altitude), climate (mean annual temperature, MAT; mean annual precipitation, MAP; growing season temperature, GST; growing season precipitation, GSP; potential evapotranspiration, PET; and actual evapotranspiration, AET) and site descriptions (vegetation and soil types). The data sets are unique because they integrate plant above- and belowground traits, climate, and soil factors over broad regional, elevational, and taxonomic ranges in understudied regions (e.g., the Tibetan Plateau). This is the only database on China's grassland species for unrestricted global access. These data sets will make a valuable contribution to future large-scale trait-based ecological studies.© 2017 by the Ecological Society of America.
Tissue analysis as a measure of nutrient availability for the growth of angiosperm aquatic plants
DOI:10.4319/lo.1966.11.4.0529 URL [本文引用: 1]
Growth rate influence on the chemical composition of phytoplankton in oceanic waters
DOI:10.1038/279210a0 URL [本文引用: 1]
Nutrient concentrations in fine roots
DOI:10.1890/0012-9658(2000)081[0275:NCIFR]2.0.CO;2 URL [本文引用: 1]
N:P ratios in terrestrial plants: variation and functional significance
DOI:10.1111/j.1469-8137.2004.01192.x
PMID:33873556
[本文引用: 6]
Nitrogen (N) and phosphorus (P) availability limit plant growth in most terrestrial ecosystems. This review examines how variation in the relative availability of N and P, as reflected by N : P ratios of plant biomass, influences vegetation composition and functioning. Plastic responses of plants to N and P supply cause up to 50-fold variation in biomass N : P ratios, associated with differences in root allocation, nutrient uptake, biomass turnover and reproductive output. Optimal N : P ratios - those of plants whose growth is equally limited by N and P - depend on species, growth rate, plant age and plant parts. At vegetation level, N : P ratios <10 and >20 often (not always) correspond to N- and P-limited biomass production, as shown by short-term fertilization experiments; however long-term effects of fertilization or effects on individual species can be different. N : P ratios are on average higher in graminoids than in forbs, and in stress-tolerant species compared with ruderals; they correlate negatively with the maximal relative growth rates of species and with their N-indicator values. At vegetation level, N : P ratios often correlate negatively with biomass production; high N : P ratios promote graminoids and stress tolerators relative to other species, whereas relationships with species richness are not consistent. N : P ratios are influenced by global change, increased atmospheric N deposition, and conservation managment. Contents Summary 243 I Introduction 244 II Variability of N : P ratios in response to nutrient supply 244 III Critical N : P ratios as indicators of nutrient limitation 248 IV Interspecific variation in N : P ratios 252 V Vegetation properties in relation to N : P ratios 255 VI Implications of N : P ratios for human impacts on ecosystems 258 VII Conclusions 259 Acknowledgements 259 References 260.
Leaf nitrogen and phosphorus stoichiometry across 753 terrestrial plant species in China
DOI:10.1111/nph.2005.168.issue-2 URL [本文引用: 5]
Biogeography and variability of eleven mineral elements in plant leaves across gradients of climate, soil and plant functional type in China
DOI:10.1111/j.1461-0248.2011.01641.x
PMID:21692962
[本文引用: 9]
Understanding variation of plant nutrients is largely limited to nitrogen and to a lesser extent phosphorus. Here we analyse patterns of variation in 11 elements (nitrogen/phosphorus/potassium/calcium/magnesium/sulphur/silicon/iron/sodium/manganese/aluminium) in leaves of 1900 plant species across China. The concentrations of these elements show significant latitudinal and longitudinal trends, driven by significant influences of climate, soil and plant functional type. Precipitation explains more variation than temperature for all elements except phosphorus and aluminium, and the 11 elements differentiate in relation to climate, soil and functional type. Variability (assessed as the coefficient of variation) and environmental sensitivity (slope of responses to environmental gradients) are lowest for elements that are required in the highest concentrations, most abundant and most often limiting in nature (the Stability of Limiting Elements Hypothesis). Our findings can help initiate a more holistic approach to ecological plant nutrition and lay the groundwork for the eventual development of multiple element biogeochemical models.© 2011 Blackwell Publishing Ltd/CNRS.
Relationship between the relative limitation and resorption efficiency of nitrogen vs phosphorus in woody plants
DOI:10.1371/journal.pone.0083366 URL [本文引用: 8]
Untangling the influence of phylogeny, soil and climate on leaf element concentrations in a biodiversity hotspot
DOI:10.1111/fec.2015.29.issue-2 URL [本文引用: 1]
Ecology of coarse woody debris in temperate ecosystems
Chemical composition of certain aquatic plants
DOI:10.1086/334455 URL [本文引用: 1]
Ecological stoichiometry of N and P in pelagic ecosystems: comparison of lakes and oceans with emphasis on the zooplankton-phytoplankton interaction
DOI:10.4319/lo.1997.42.4.0648 URL [本文引用: 1]
Stoichiometry and large-scale patterns of leaf carbon and nitrogen in the grassland biomes of China
DOI:10.1007/s00442-006-0425-0 URL [本文引用: 3]
Ecological stoichiometry: searching for unifying principles from individuals to ecosystems
生态化学计量学: 探索从个体到生态系统的统一化理论
DOI:10.3773/j.issn.1005-264x.2010.01.002 [本文引用: 2]
Leaf nitrogen:phosphorus stoichiometry across Chinese grassland biomes
DOI:10.1007/s00442-007-0912-y URL [本文引用: 1]
Taxonomic identity, phylogeny, climate and soil fertility as drivers of leaf traits across Chinese grassland biomes
DOI:10.1007/s10265-009-0294-9 URL
A test of the generality of leaf trait relationships on the Tibetan Plateau
DOI:10.1111/nph.2006.170.issue-4 URL [本文引用: 1]
Carbon, nitrogen and phosphorus stoichiometry in the coarse roots of 45 desert plant species in relation to environmental factors across the deserts in Xinjiang
新疆45种荒漠植物粗根碳、氮、磷计量特征及其与环境的关系
Drought effect on plant nitrogen and phosphorus: a meta-analysis
DOI:10.1111/nph.2014.204.issue-4 URL [本文引用: 1]
Leaf nitrogen and phosphorus of temperate desert plants in response to climate and soil nutrient availability
DOI:10.1038/srep06932 URL [本文引用: 1]
Influence of life form, taxonomy, climate, and soil properties on shoot and root concentrations of 11 elements in herbaceous plants in a temperate desert
DOI:10.1007/s11104-015-2669-0 URL [本文引用: 1]
Divergent variations in concentrations of chemical elements among shrub organs in a temperate desert
DOI:10.1038/srep20124 URL [本文引用: 1]
Nutrient levels within leaves, stems, and roots of the xeric species Reaumuria soongorica in relation to geographical, climatic, and soil conditions
DOI:10.1002/ece3.2015.5.issue-7 URL [本文引用: 2]
Plant trait networks: improved resolution of the dimensionality of adaptation
DOI:10.1016/j.tree.2020.06.003 URL [本文引用: 1]
Ecosystem traits linking functional traits to macroecology
DOI:10.1016/j.tree.2018.11.004 URL [本文引用: 1]
Patterns and influencing factors of traits in forest ecosystems: synthesis and perspectives on the synthetic investigation from the north-east transect of eastern China (NETEC)
森林生态系统性状的空间格局与影响因素研究进展--基于中国东部样带的整合分析
The biogeochemical niche shifts of Pinus sylvestris var. mongolica along an environmental gradient
DOI:10.1016/j.envexpbot.2019.103825 URL [本文引用: 1]
RNA responses to N- and P-limitation: reciprocal regulation of stoichiometry and growth rate in Brachionus
DOI:10.1111/fec.2007.21.issue-5 URL [本文引用: 1]
Growth, chlorophyll and mineral nutrient studies on Phalaris arundinacea L. in three Scottish lochs
DOI:10.1007/BF00021014 URL [本文引用: 1]
Inorganic mineral nutrient level studies on Potamogeton pectinatus L. and Enteromorpha prolifera in Forfar Loch, Scotland
DOI:10.1007/BF00012558 URL [本文引用: 1]
Tree growth and soil acidification in response to 30 years of experimental nitrogen loading on boreal forest
DOI:10.1111/gcb.2006.12.issue-3 URL [本文引用: 1]
Stoichiometry of root and leaf nitrogen and phosphorus in a dry alpine steppe on the Northern Tibetan Plateau
DOI:10.1371/journal.pone.0109052 URL
Tissue nutrient content of Gracilaria spp. (Rhodophyta) and water quality along an estuarine gradient
DOI:10.1071/MF9950975 URL [本文引用: 1]
Macro element nutrition of pine, spruce, and birch seedlings in nutrient solutions
A definition of optimum nutrient requirements in birch seedlings. I.
DOI:10.1111/ppl.1970.23.issue-6 URL [本文引用: 1]
A definition of optimum nutrient requirements in birch seedlings. II.
DOI:10.1111/ppl.1971.24.issue-1 URL [本文引用: 1]
Mineral nutrient requirements of Pinus silvestris and Picea abies seedlings
DOI:10.1111/ppl.1979.45.issue-4 URL [本文引用: 1]
Empirical relationships between the element composition of aquatic macrophytes and their underlying sediments
A global budget for fine root biomass, surface area, and nutrient contents
Global biogeochemical models have improved dramatically in the last decade in their representation of the biosphere. Although leaf area data are an important input to such models and are readily available globally, global root distributions for modeling water and nutrient uptake and carbon cycling have not been available. This analysis provides global distributions for fine root biomass, length, and surface area with depth in the soil, and global estimates of nutrient pools in fine roots. Calculated root surface area is almost always greater than leaf area, more than an order of magnitude so in grasslands. The average C:N:P ratio in living fine roots is 450:11:1, and global fine root carbon is more than 5% of all carbon contained in the atmosphere. Assuming conservatively that fine roots turn over once per year, they represent 33% of global annual net primary productivity.
Beyond macronutrients: element variability and multielement stoichiometry in freshwater invertebrates
DOI:10.1111/ele.2006.9.issue-12 URL [本文引用: 1]
TRY-A global database of plant traits
Phylogenetic and growth form variation in the scaling of nitrogen and phosphorus in the seed plants
The terminological jungle revisited: making a case for use of the term resorption
DOI:10.2307/3565477 URL [本文引用: 1]
The vegetation N:P ratio: a new tool to detect the nature of nutrient limitation
DOI:10.2307/2404783 URL [本文引用: 10]
The stoichiometry of animal energetics
DOI:10.1006/jtbi.1995.0232 URL [本文引用: 1]
The nutritional status of plants from high altitudes
DOI:10.1007/BF00377088
PMID:28311193
[本文引用: 1]
Are plants at high altitudes short in nutrients? In order to answer this question the mineral nutrient content of leaves from over 150 plant species from 9 different mountain areas of all major climatic zones were analyzed (Kjeldahl nitrogen in all, phosphate in half of the samples, K, Mg, Mn, Ca in the Alps only). The majority of data are from herbaceous perennials, but shrubs and trees were studied as well. N-partitioning was studied in 45 herbaceous species from contrasting altitudes in the Alps. The survey falls into three categories: (1) comparisons of whole communities of species from contrasting altitudes, (2) analysis of altitudinal gradients, and (3) additional collections from high altitude sites alone. Unlike the other mineral nutrients, nitrogen content follows consistent altitudinal and latitudinal trends. The higher altitude sample always had higher N content per unit leaf area, irrespective of life form, wherever comparable plants (the same or related species) were investigated at contrasting altitudes. N content per unit dry weight (%) increased with altitude in herbaceous plants (in some species >4%), but was remarkably stable in evergreen woody plants (around 1%). The mean fraction of total plant N allocated to leaves of herbaceous plants in the Alps was the same at low and high altitude (1/3 of total). Leaf N (%) from the regional upper limits of higher plant life reveals a latitudinal decrease from subarctic to equatorial mountains, which may be related to the duration of annual leaf activity. Since mean N content per leaf area hardly differs between the uppermost sites, life span expectation (sink-duration) seems to control carbon investments rather than N input per leaf area. The growth of leaves at high altitude seems to be controlled in a way that leads to comparatively high nutrient contents, which in turn support high metabolic activity. Inherent developmental growth constraints inhibit nutrient dilution in the plant body and thus defy the application of classical concepts of plant-nutrient versus soil-nutrient relations developed for lowlands and in particular for cultivated plants. The results re-emphasize the global significance of links between nitrogen content, leaf sclerophylly, leaf longevity and photosynthetic capacity.
Inherent variation in growth rate between higher plants: a search for physiological causes and ecological consequences
The mineral nutrient requirements of forest trees
Nitrogen and phosphorus allocation in leaves, twigs, and fine roots across 49 temperate, subtropical and tropical tree species: a hierarchical pattern
DOI:10.1111/fec.2010.24.issue-1 URL [本文引用: 1]
Nitrogen deposition and its ecological impact in China: an overview
DOI:10.1016/j.envpol.2010.08.002 URL [本文引用: 1]
The origins of the Redfield nitrogen-to-phosphorus ratio are in a homoeostatic protein-to-rRNA ratio
DOI:10.1111/j.1461-0248.2010.01577.x
PMID:21244593
[本文引用: 1]
One of the most intriguing patterns in the biosphere is the similarity of the atomic nitrogen-to-phosphorus ratio (N:P) = 16 found in waters throughout the deep ocean and in the plankton in the upper ocean. Although A.C. Redfield proposed in 1934 that the intracellular properties of plankton were central to this pattern, no theoretical significance for N:P = 16 in cells had been found. Here, we use theoretical modelling and a compilation of literature data for prokaryotic and eukaryotic microbes to show that the balance between two fundamental processes, protein and rRNA synthesis, results in a stable biochemical attractor that homoeostatically produces a given protein:rRNA ratio. Furthermore, when biochemical constants and reasonable kinetic parameters for protein synthesis and ribosome production under nutrient-replete conditions are applied in the model, it predicts a stable protein:rRNA ratio of 3 ± 0.7, which corresponds to N:P = 16 ± 3. The model also predicts that N-limitation, by constraining protein synthesis rates, will result in N:P ratios below the Redfield value while P-limitation, by constraining RNA production rates, will produce ratios above the Redfield value. Hence, one of most biogeochemically significant patterns on Earth is inherently rooted in the fundamental structure of life.© 2011 Blackwell Publishing Ltd/CNRS.
N, P and K stoichiometry and resorption efficiency of nine dominant shrub species in the deserts of Xinjiang, China
DOI:10.1111/ere.v35.4 URL [本文引用: 1]
Patterns of nitrogen and phosphorus stoichiometry among leaf, stem and root of desert plants and responses to climate and soil factors in Xinjiang, China
DOI:10.1016/j.catena.2020.105100 URL [本文引用: 1]
Spatial changes and influencing factors of fine root carbon, nitrogen and phosphorus stoichiometry of plants in China
DOI:10.17521/cjpe.2015.0015 URL [本文引用: 1]
中国植物细根碳、氮、磷化学计量学的空间变化及其影响因子
DOI:10.17521/cjpe.2015.0015
[本文引用: 1]
为了研究中国陆地植物细根碳(C)、氮(N)、磷(P)的空间变化模式, 揭示细根在“温度-植物生理假说”及“生长速率假说”等方面的规律, 该文收集已发表的有关中国陆地植物细根研究的文献, 从中提取细根C、N、P元素含量及其相关数据, 分析了细根C、N、P含量及其比例与经纬度之间的关系。结果表明: 细根N、P元素含量均随纬度增加而增加, P含量随经度增加而降低, N:P随经度增加而增加。细根N、P含量与年平均气温、年降水量均呈负相关关系, 与土壤养分呈正相关关系。在土壤养分、温度、降水量3个非生物因素中, 土壤养分对细根N、P含量的影响最大。该文中细根和粗根的C:P、N:P差异变化不完全支持“生长速率假说”。根系和叶片一样, N、P含量与纬度呈正相关关系, 支持“温度-植物生理假说”, 反映了植物对自然环境的适应策略。
Are bacteria more like plants or animals? Growth rate and resource dependence of bacterial C:N:P stoichiometry
DOI:10.1046/j.1365-2435.2003.00712.x URL [本文引用: 1]
Importance of cycling and recycling of mineral nutrients within plants for growth and development
DOI:10.1111/plb.1997.110.issue-4 URL [本文引用: 1]
N:P stoichiometry and protein:RNA ratios in vascular plants: an evaluation of the growth-rate hypothesis
DOI:10.1111/ele.2009.12.issue-8 URL [本文引用: 1]
Species-specific responses of foliar nutrients to long-term nitrogen and phosphorus additions in a lowland tropical forest
DOI:10.1111/jec.2013.102.issue-1 URL [本文引用: 2]
Scaling of C:N:P stoichiometry in forests worldwide: implications of terrestrial Redfield-type ratios
DOI:10.1890/03-0351 URL [本文引用: 2]
Mineral and nitrogen content of the leaves of some forest trees at different times in the growing season
DOI:10.1086/334303 URL [本文引用: 1]
Mineral nutrient concentrations in sapwood and heartwood: a literature review
DOI:10.1051/forest:2002059 URL [本文引用: 1]
Leaf chemistry of woody species in the Brazilian cerrado and seasonal forest: response to soil and taxonomy and effects on decomposition rates
DOI:10.1007/s11258-016-0658-x URL [本文引用: 1]
Nitrogen addition reduces soil respiration in a mature tropical forest in southern China
DOI:10.1111/j.1365-2486.2007.01503.x URL [本文引用: 1]
Phosphorus deficiency induced by nitrogen input in Douglas fir in the Netherlands
DOI:10.1007/BF02375071 URL [本文引用: 1]
Plant allometry, leaf nitrogen and phosphorus stoichiometry, and interspecific trends in annual growth rates
Life forms as diverse as unicellular algae, zooplankton, vascular plants, and mammals appear to obey quarter-power scaling rules. Among the most famous of these rules is Kleiber's (i.e. basal metabolic rates scale as the three-quarters power of body mass), which has a botanical analogue (i.e. annual plant growth rates scale as the three-quarters power of total body mass). Numerous theories have tried to explain why these rules exist, but each has been heavily criticized either on conceptual or empirical grounds. N,P-STOICHIOMETRY: Recent models predicting growth rates on the basis of how total cell, tissue, or organism nitrogen and phosphorus are allocated, respectively, to protein and rRNA contents may provide the answer, particularly in light of the observation that annual plant growth rates scale linearly with respect to standing leaf mass and that total leaf mass scales isometrically with respect to nitrogen but as the three-quarters power of leaf phosphorus. For example, when these relationships are juxtaposed with other allometric trends, a simple N,P-stoichiometric model successfully predicts the relative growth rates of 131 diverse C3 and C4 species.The melding of allometric and N,P-stoichiometric theoretical insights provides a robust modelling approach that conceptually links the subcellular 'machinery' of protein/ribosomal metabolism to observed growth rates of uni- and multicellular organisms. Because the operation of this 'machinery' is basic to the biology of all life forms, its allometry may provide a mechanistic explanation for the apparent ubiquity of quarter-power scaling rules.
N, P, and C stoichiometry of Eranthis hyemalis (Ranunculaceae) and the allometry of plant growth
DOI:10.3732/ajb.92.8.1256
PMID:21646146
[本文引用: 5]
We report the nitrogen (N), phosphorus (P), and carbon (C) stoichiometry for each of the five organ-types (leaves, aerial stems, reproductive organs, roots, and tubers) of 17 actively growing Eranthis hyemalis plants differing in size (as measured in g C). We also report the N, P, and C stoichiometry of 20 winterized tubers, which are the only perennial organs of this species. Comparisons between whole-plant and winterized N/C and P/C levels indicate that N was resorbed from aerial organs and stored in tubers by the end of the growing season. Leaves were substantial reservoirs for N and P. With few exceptions, N scaled isometrically with respect to C for each organ-type, whereas P scaled as the 3/4 power of C. Thus, N is proportional to P(3/4), which is proportional to C regardless of organ-type. Additionally, annual growth rate G of shoots (leaves and aerial stems) scaled as the -3 power of leaf N/P quotients such that G was proportional to the 3/4 power of leaf P. We suggest that these scaling relationships (together with previously reported allometric trends across herbaceous species) show that growth is constrained by organ-specific N and P allocation patterns (presumably to proteins and ribosomes, respectively).
Nitrogen/phosphorus leaf stoichiometry and the scaling of plant growth
DOI:10.1111/ele.2008.8.issue-6 URL [本文引用: 5]
Nitrogen absorption by roots as a cause of interspecific variations in leaf nitrogen concentration and photosynthetic capacity
DOI:10.1111/fec.2005.19.issue-3 URL [本文引用: 1]
Foliar nitrogen and phosphorus accumulation responses after fertilization: an example from nutrient-limited Hawaiian forests
DOI:10.1007/s11104-010-0281-x URL [本文引用: 1]
N and P in New Zealand soil chronosequences and relationships with foliar N and P.
DOI:10.1007/s10533-004-7790-8 URL [本文引用: 1]
Phytoplankton in the English lakes: II. The composition of the phytoplankton in relation to dissolved substances
DOI:10.2307/2256077 URL [本文引用: 1]
Stage-dependent stoichiometric homeostasis and responses of nutrient resorption in Amaranthus mangostanus to nitrogen and phosphorus addition
DOI:10.1038/srep37219 URL [本文引用: 2]
The bioelements, the elementome, and the biogeochemical niche
DOI:10.1002/ecy.2652 URL [本文引用: 6]
Human-induced nitrogen-phosphorus imbalances alter natural and managed ecosystems across the globe
DOI:10.1038/ncomms3934
PMID:24343268
[本文引用: 1]
The availability of carbon from rising atmospheric carbon dioxide levels and of nitrogen from various human-induced inputs to ecosystems is continuously increasing; however, these increases are not paralleled by a similar increase in phosphorus inputs. The inexorable change in the stoichiometry of carbon and nitrogen relative to phosphorus has no equivalent in Earth's history. Here we report the profound and yet uncertain consequences of the human imprint on the phosphorus cycle and nitrogen: phosphorus stoichiometry for the structure, functioning and diversity of terrestrial and aquatic organisms and ecosystems. A mass balance approach is used to show that limited phosphorus and nitrogen availability are likely to jointly reduce future carbon storage by natural ecosystems during this century. Further, if phosphorus fertilizers cannot be made increasingly accessible, the crop yields projections of the Millennium Ecosystem Assessment imply an increase of the nutrient deficit in developing regions.
Faster returns on “leaf economics” and different biogeochemical niche in invasive compared with native plant species
DOI:10.1111/gcb.2010.16.issue-8 URL [本文引用: 2]
Nutrient stoichiometric relations and biogeochemical niche in coexisting plant species: effect of simulated climate change
The human-induced imbalance between C, N and P in Earth’s life system
DOI:10.1111/j.1365-2486.2011.02568.x URL [本文引用: 1]
The biological control of chemical factors in the environment
Stoichiometric patterns in foliar nutrient resorption across multiple scales
DOI:10.1111/nph.2012.196.issue-1 URL [本文引用: 1]
Relationships among RNA:DNA ratio, growth and elemental stoichiometry in mangrove trees
DOI:10.1111/j.1365-2435.2010.01722.x URL [本文引用: 1]
Global patterns of plant leaf N and P in relation to temperature and latitude
Leaf phosphorus influences the photosynthesis-nitrogen relation: a cross-biome analysis of 314 species
DOI:10.1007/s00442-009-1291-3 URL [本文引用: 2]
Evidence of a general 2/3-power law of scaling leaf nitrogen to phosphorus among major plant groups and biomes
DOI:10.1098/rspb.2009.1818 URL [本文引用: 6]
Photosynthesis-nitrogen relations in Amazonian tree species I. Patterns among species and communities
DOI:10.1007/BF00317909
PMID:28313590
[本文引用: 1]
Among species, photosynthetic capacity (A) is usually related to leaf nitrogen content (N), but variation in the species-specific relationship is not well understood. To address this issue, we studied A-N relationships in 23 species in adjacent Amazonian communities differentially limited by nitrogen (N), phosphorus (P), and/or other mineral nutrients. Five species were studied in each of three late successional forest types (Tierra Firme, Caatinga and Bana) and eight species were studied on disturbed sites (cultivated and early secondary successional Tierra Firme plots). A expressed on a mass basis (A) was correlated (p<0.05) with N in 17 of 23 species, and A on an area basis (A) was correlated (p<0.05) with N in 21 of 23 species. The slopes of A-N relationships were greater and intercepts lower for disturbance adapted early successional species than for late successional species. On a mass basis, the A-N slope averaged ≈15 μmol CO [g N] s for 7 early secondary successional species and ≈4 μmol CO [g N] s for 15 late successional species, respectively. Species from disturbed sites had shorter leaf life-span and greater specific leaf area (SLA) than late successional species. Across all 23 species, the slope of the A-N relationship was related (p<0.001) positively to SLA (r=0.70) and negatively to leaf life-span (r=0.78) and temporal niche during secondary succession (years since cutting-and-burning, r=0.90). Thus, disturbance adapted early successional species display a set of traits (short leaf life-span, high SLA and A and a steep slope of A-N) conducive to resource acquisition and rapid growth in their high resource regeneration niches. The significance and form of the A-N relationship were associated with the relative nutrient limitations in the three late successional communities. At species and community levels, A was more highly dependent on N in the N-limited Caatinga than in the P-and N-limited Bana and least in the P-and Ca-limited Tierra Firme on oxisol-and differences among these three communities in their massbased A-N slope reflects this pattern (6.0, 2.4, and 0.7 μmol CO [g N] s, respectively). Among all 23 species, the estimated leaf N needed to reach compensation (net photosynthesis ≈ zero) was positively related to the A-N slope and to dark respiration rates and negatively related to leaf life-span. Variation among species in the A-N slope was well correlated with potential photosynthetic N use efficiency, A per unit leaf N. The dependence of A on N and the form of the relationship vary among Amazonian species and communities, consistent with both relative availabilities of N, P, and other mineral nutrients, and with intrinsic ecophysiological characteristics of species adapted to habitats of varying resource availability.
Different photosynthesis-nitrogen relations in deciduous hardwood and evergreen coniferous tree species
DOI:10.1007/BF00365558
PMID:28306909
[本文引用: 1]
The relationship between photosynthetic capacity (A ) and leaf nitrogen concentration (N) among all C species can be described roughly with one general equation, yet within that overall pattern species groups or individual species may have markedly different A -N relationships. To determine whether one or several predictive, fundamental A -N relationships exist for temperate trees we measured A, specific leaf area (SLA) and N in 22 broad-leaved deciduous and 9 needle-leaved evergreen tree species in Wisconsin, United States. For broad-leaved deciduous trees, mass-based A was highly correlated with leaf N (r =0.75, P<0.001). For evergreen conifers, mass-based A was also correlated with leaf N (r =0.59, P<0.001) and the slope of the regression (rate of increase of A per unit increase in N) was lower (P<0.001) by two-thirds than in the broad-leaved species (1.9 vs. 6.4 μmol CO g N s), consistent with predictions based on tropical rain forest trees of short vs. long leaf life-span. On an area basis, there was a strong A -N correlation among deciduous species (r =0.78, P<0.001) and no correlation (r =0.03, P>0.25) in the evergreen conifers. Compared to deciduous trees at a common leaf N (mass or area basis), evergreen trees had lower A and SLA. For all data pooled, both leaf N and A on a mass basis were correlated (r =0.6) with SLA; in contrast, area-based leaf N scaled tightly with SLA (r =0.81), but area-based A did not (r =0.06) because of low A per unit N in the evergreen conifers. Multiple regression analysis of all data pooled showed that both N (mass or area basis) and SLA were significantly (P<0.001) related to A on mass (r =0.80) and area (r =0.55) bases, respectively. These results provide further evidence that A -N relationships are fundamentally different for ecologically distinct species groups with differing suites of foliage characteristics: species with long leaf life-spans and low SLA, whether broad-leaved or needle-leaved, tend to have lower A per unit leaf N and a lower slope and higher intercept of the A -N relation than do species with shorter leaf life-span and higher SLA. A single global A -N equation overestimates and underestimates A for temperate trees at the upper and lower end of their leaf N range, respectively. Users of A -N relationships in modeling photosynthesis in different ecosystems should appreciate the strengths and limitations of regression equations based on different species groupings.
Leaf nitrogen and phosphorus stoichiometry across 654 terrestrial plant species in NSTEC
中国东部南北样带654种植物叶片氮和磷的化学计量学特征研究
Optimum N:P ratios and coexistence of planktonic algae
DOI:10.1111/jpy.1980.16.issue-4 URL [本文引用: 1]
A survey of the chemical composition of Potamogeton and Myriophyllum in New Jersey
DOI:10.1017/S0043174500031362 URL [本文引用: 1]
Nitrogen, phosphorus, and eutrophication in the coastal marine environment
The distribution of inorganic nitrogen and phosphorus and bioassay experiments both show that nitrogen is the critical limiting factor to algal growth and eutrophication in coastal marine waters. About twice the amount of phosphate as can be used by the algae is normally present. This surplus results from the low nitrogen to phosphorus ratio in terrigenous contributions, including human waste, and from the fact that phosphorus regenerates more quickly than ammonia from decomposing organic matter. Removal of phosphate from detergents is therefore not likely to slow the eutrophication of coastal marine waters, and its replacement with nitrogen-containing nitrilotriacetic acid may worsen the situation.
Nitrogen content in fine roots and the structural and functional adaptations of alpine plants
DOI:10.1134/S2079086414030074 URL
Changes in nutrient concentrations of leaves and roots in response to global change factors
DOI:10.1111/gcb.13721
PMID:28407324
[本文引用: 1]
Global change impacts on biogeochemical cycles have been widely studied, but our understanding of whether the responses of plant elemental composition to global change drivers differ between above- and belowground plant organs remains incomplete. We conducted a meta-analysis of 201 reports including 1,687 observations of studies that have analyzed simultaneously N and P concentrations changes in leaves and roots in the same plants in response to drought, elevated [CO ], and N and P fertilization around the world, and contrasted the results within those obtained with a general database (838 reports and 14,772 observations) that analyzed the changes in N and P concentrations in leaves and/or roots of plants submitted to the commented global change drivers. At global level, elevated [CO ] decreased N concentrations in leaves and roots and decreased N:P ratio in roots but no in leaves, but was not related to P concentration changes. However, the response differed among vegetation types. In temperate forests, elevated [CO ] was related with lower N concentrations in leaves but not in roots, whereas in crops, the contrary patterns were observed. Elevated [CO ] decreased N concentrations in leaves and roots in tundra plants, whereas not clear relationships were observed in temperate grasslands. However, when elevated [CO ] and N fertilization coincided, leaves had lower N concentrations, whereas root had higher N concentrations suggesting that more nutrients will be allocated to roots to improve uptake of the soil resources not directly provided by the global change drivers. N fertilization and drought increased foliar and root N concentrations while the effects on P concentrations were less clear. The changes in N and P allocation to leaves and root, especially those occurring in opposite direction between them have the capacity to differentially affect above- and belowground ecosystem functions, such as litter mineralization and above- and belowground food webs.© 2017 John Wiley & Sons Ltd.
Foliar elemental composition of European forest tree species associated with evolutionary traits and present environmental and competitive conditions
DOI:10.1111/geb.2015.24.issue-2 URL [本文引用: 1]
Tree growth changes with climate and forest type are associated with relative allocation of nutrients, especially phosphorus, to leaves and wood
DOI:10.1111/geb.12015 URL [本文引用: 1]
Climate and taxonomy underlie different elemental concentrations and stoichiometries of forest species: the optimum “biogeochemical niche”
We previously hypothesised the existence of a "biogeochemical niche" occupied by each plant species. Different species should have a specific elemental composition, stoichiometry and allocation as a consequence of their particular metabolism, physiology and structure (morphology) linked to their optimal functioning under the environmental (abiotic and biotic) conditions where they have evolved. We tested this hypothesis using data from the Catalan Forestry Inventory that covers different forest groups growing under a large climatic gradient. Mediterranean species that occupy hotter-drier environments have lower leaf N, P and K concentrations than non-Mediterranean forest species. Within a determined climatic biome, different species competing in the same space have different elemental compositions and allocations linked to their taxonomical differences and their phenotypic plasticity. Gymnosperms have a proportionally higher elemental allocation to leaves than to wood, higher C concentrations, and lower N, P and K concentrations mainly in the stem and branches than angiosperms. The differences among species are linked to asymmetrical use of different elements, suggesting that the biogeochemical niche is a final expression and consequence of long-term species adaptation to particular abiotic factors, ecological role (stress tolerant, ruderal, competitor), different soil occupation and use of resources to avoid interspecific competition, and finally of a certain degree of flexibility to adapt to current environmental shifts.
Factors affecting nutrient concentration and stoichiometry of forest trees in Catalonia (NE Spain)
DOI:10.1016/j.foreco.2011.08.019 URL [本文引用: 1]
The C:N:P stoichiometry of organisms and ecosystems in a changing world: a review and perspectives
DOI:10.1016/j.ppees.2011.08.002 URL [本文引用: 5]
Empirical support for the biogeochemical niche hypothesis in forest trees
Stem, root, and older leaf N:P ratios are more responsive indicators of soil nutrient availability than new foliage
Foliar nitrogen to phosphorus (N:P) ratios are widely used to indicate soil nutrient availability and limitation, but the foliar ratios of woody plants have proven more complicated to interpret than ratios from whole biomass of herbaceous species. This may be related to tissues in woody species acting as nutrient reservoirs during active growth, allowing maintenance of optimal N:P ratios in recently produced, fully expanded leaves (i.e., "new" leaves, the most commonly sampled tissue). Here we address the hypothesis that N:P ratios of newly expanded leaves are less sensitive indicators of soil nutrient availability than are other tissue types in woody plants. Seedlings of five naturally established tree species were harvested from plots receiving two years of fertilizer treatments in a lowland tropical forest in the Republic of Panama. Nutrient concentrations were determined in new leaves, old leaves, stems, and roots. For stems and roots, N:P ratios increased after N addition and decreased after P addition, and trends were consistent across all five species. Older leaves also showed strong responses to N and P addition, and trends were consistent for four of five species. In comparison, overall N:P ratio responses in new leaves were more variable across species. These results indicate that the N:P ratios of stems, roots, and older leaves are more responsive indicators of soil nutrient availability than are those of new leaves. Testing the generality of this result could improve the use of tissue nutrient ratios as indices of soil nutrient availability in woody plants.
Nutrient budgets of marsh plants: efficiency concepts and relation to availability
DOI:10.2307/1939129 URL [本文引用: 1]
Stoichiometry and chemical metrology: Karl Fischer reaction
DOI:10.1007/s007690050357 URL [本文引用: 1]
Stoichiometric flexibility in response to fertilization along gradients of environmental and organismal nutrient richness
DOI:10.1111/oik.2015.v124.i7 URL [本文引用: 2]
Stoichiometric flexibility as a regulator of carbon and nutrient cycling in terrestrial ecosystems under change
DOI:10.1111/j.1469-8137.2012.04234.x
PMID:22924404
[本文引用: 2]
Ecosystems across the biosphere are subject to rapid changes in elemental balance and climatic regimes. A major force structuring ecological responses to these perturbations lies in the stoichiometric flexibility of systems - the ability to adjust their elemental balance whilst maintaining function. The potential for stoichiometric flexibility underscores the utility of the application of a framework highlighting the constraints and consequences of elemental mass balance and energy cycling in biological systems to address global change phenomena. Improvement in the modeling of ecological responses to disturbance requires the consideration of the stoichiometric flexibility of systems within and across relevant scales. Although a multitude of global change studies over various spatial and temporal scales exist, the explicit consideration of the role played by stoichiometric flexibility in linking micro-scale to macro-scale biogeochemical processes in terrestrial ecosystems remains relatively unexplored. Focusing on terrestrial systems under change, we discuss the mechanisms by which stoichiometric flexibility might be expressed and connected from organisms to ecosystems. We suggest that the transition from the expression of stoichiometric flexibility within individuals to the community and ecosystem scales is a key mechanism regulating the extent to which environmental perturbation may alter ecosystem carbon and nutrient cycling dynamics.© 2012 The Authors. New Phytologist © 2012 New Phytologist Trust.
Annual changes of nitrogen and phosphorus in two aquatic macrophytes (Nymphaea tuberosa and Ceratophyllum demersum)
DOI:10.1007/BF00015487 URL [本文引用: 1]
Direct estimation of aboveground forest productivity through hyperspectral remote sensing of canopy nitrogen
DOI:10.1890/1051-0761(2002)012[1286:DEOAFP]2.0.CO;2 URL [本文引用: 1]
The nitrogen and phosphorus dependence of algal biomass in lakes: an empirical and theoretical analysis
DOI:10.4319/lo.1982.27.6.1101 URL [本文引用: 1]
A meta-analysis of 1,119 manipulative experiments on terrestrial carbon-cycling responses to global change
Responses of δ13C value and water use efficiency of plant species to environmental gradients along the grassland zone of northeast China transect
中国东北样带草原区植物δ13C值及水分利用效率对环境梯度的响应
测定了中国东北森林—草原样带草原区15个常见植物种叶片的δ13C值,并以此作为植物长期水分利用效率的指示值,研究了不同植物种的水分利用效率对年均降水量、年均大气温度和海拔高度等环境梯度变化的响应。结果表明:有相当一部分植物种的δ13C值和水分利用效率均随年均降水量和年均温增加而呈不同程度的降低趋势(如羊草(Leymus chinensis(Trin.)Tzvel.)、家榆(Ulmus pumila L.)、小叶锦鸡儿(Caragana microphylla Lam.)、直立黄芪(Astragalus adsurgens Pall.)、地榆(Sanguisorba officinalis L.)和菊叶委陵菜(Potentila tanacetifolia Willd.ex Schlecht.)等),随海拔高度增高而呈不同程度的增加趋势(如扁蓿豆(Melissitus ruthenicus(L.)Peschkova)、羊草、家榆、小叶锦鸡儿、直立黄芪、地榆等):而少数几个种(如达乌里胡枝子(Lespedeza davurica(Laxm.)Schindl.),麻花头(Serratula centauroide L.)等)则与大多数种的情况截然相反,另外部分植物种则随环境因子变化不大(达乌里黄芪(Astragalus dahuricus (Pall.)DC.)、中间锦鸡儿(Caragana intermedia Kuang et H.C.Fu)和狭叶锦鸡儿(Caragana stenophylla Pojark.),冷蒿(Artemisia frigida Willd.)、糙叶黄芪(Astragalus scaberrimus Bunge)、甘草(Glycyrrhiza uralensis Fisch.)等)。这表明,不同植物种的水分利用状况对环境梯度变化的响应不同,不同植物种具有不同的适应环境变化的策略。在退化草地生态系统恢复的实践中,应该选择具有较强适应干旱环境的植物种作为恢复物种。
Biogeographic patterns of nutrient resorption from Quercus variabilis Blume leaves across China
DOI:10.1111/plb.12420
PMID:26597338
[本文引用: 1]
The variation in nutrient resorption has been studied at different taxonomic levels and geographic ranges. However, the variable traits of nutrient resorption at the individual species level across its distribution are poorly understood. We examined the variability and environmental controls of leaf nutrient resorption of Quercus variabilis, a widely distributed species of important ecological and economic value in China. The mean resorption efficiency was highest for phosphorus (P), followed by potassium (K), nitrogen (N), sulphur (S), magnesium (Mg) and carbon (C). Resorption efficiencies and proficiencies were strongly affected by climate and respective nutrients concentrations in soils and green leaves, but had little association with leaf mass per area. Climate factors, especially growing season length, were dominant drivers of nutrient resorption efficiencies, except for C, which was strongly related to green leaf C status. In contrast, green leaf nutritional status was the primary controlling factor of leaf nutrient proficiencies, except for C. Resorption efficiencies of N, P, K and S increased significantly with latitude, and were negatively related to growing season length and mean annual temperature. In turn, N, P, K and S in senesced leaves decreased with latitude, likely due to their efficient resorption response to variation in climate, but increased for Mg and did not change for C. Our results indicate that the nutrient resorption efficiency and proficiency of Q. variabilis differed strongly among nutrients, as well as growing environments. Our findings provide important insights into understanding the nutrient conservation strategy at the individual species level and its possible influence on nutrient cycling. © 2015 German Botanical Society and The Royal Botanical Society of the Netherlands.
The birth of stoichiometry
DOI:10.1021/ed039p267 URL [本文引用: 1]
Patterns of plant carbon, nitrogen, and phosphorus concentration in relation to productivity in China’s terrestrial ecosystems
Leaf N and P stoichiometry of 57 plant species in the Karamori Mountain Ungulate Nature Reserve, Xinjiang, China
DOI:10.1007/s40333-016-0019-6 URL [本文引用: 1]
The Redfield ratio and phytoplankton growth rate
DOI:10.1017/S0025315400050566 URL [本文引用: 1]
A comparative study of leaf nutrient concentrations in a regional herbaceous flora
DOI:10.1046/j.1469-8137.1997.00787.x
PMID:33863101
[本文引用: 4]
Mineral nutrient concentrations were determined in leaves of 83 mostly herbaceous species collected from central England. Most samples were analysed for N, P, K, Ca, Mg, Na, Fe, Al, Mn, Cu and Zn. Concentrations of K, N and P showed similar levels of interspecific variability, with the highest concentrations being 6-9 times the lowest. Mg and (especially) Ca were much more variable, with the highest concentrations being 24 and 49 times the lowest respectively. Only in the case of P concentration was the majority of the variance in the data found at or below the species level. Most of the variance in Ca and Mg concentrations was between monocots and dicots. Concentrations of N and P were strongly positively correlated with each other. Only Ca and Mn were consistently associated with soil pH, positively and negatively respectively. Dicots tended to accumulate more Ca and Mn from high soil concentrations than did monocots. Concentration of P was significantly positively correlated with maximum potential relative growth rate. Plants of woodland and arable habitats contained high concentrations of P, and those of pasture and skeletal habitats contained low concentrations of P. The P: N ratio was higher in plants of arable habitats. Species with P-rich leaves tended to be currently increasing in abundance. The results suggest that plants with nutrient-rich foliage grow quickly, dominate nutrient-rich ecosystems and are generally increasing as a result of the eutrophication and disturbance arising from human exploitation.
Responses of forest ecosystems to increasing N deposition in China: a critical review
DOI:10.1016/j.envpol.2018.08.010 URL [本文引用: 1]
A global database of paired leaf nitrogen and phosphorus concentrations of terrestrial plants
DOI:10.1002/ecy.2812 [本文引用: 3]
Growth responses of trees and understory plants to nitrogen fertilization in a subtropical forest in China
DOI:10.5194/bg-14-3461-2017 URL [本文引用: 1]
Plant stoichiometry: a research frontier in ecology
植物化学计量学: 一个方兴未艾的生态学研究方向
Family-level leaf nitrogen and phosphorus stoichiometry of global terrestrial plants
DOI:10.1007/s11427-019-9584-1 URL [本文引用: 2]
Global leaf nitrogen and phosphorus stoichiometry and their scaling exponent
Plant community composition affects the species biogeochemical niche
DOI:10.1002/ecs2.1801 URL [本文引用: 1]
Reproductive allocation in Senecio sylvaticus and Chamaenerion angustifolium in relation to mineral nutrition
DOI:10.2307/2259377 URL [本文引用: 1]
Prediction of mineral nutrient status of trees by foliar analysis
DOI:10.1007/BF02860066 URL [本文引用: 1]
El inventario ecológicoy forestal de Cataluña: una herramienta para la ecologíafunctional (The ecological forest inventory of Catalonia: a tool for functional ecology)
DOI:10.7818/ECOS URL [本文引用: 1]
Global resorption efficiencies and concentrations of carbon and nutrients in leaves of terrestrial plants
DOI:10.1890/11-0416.1 URL [本文引用: 1]
Human alteration of the global nitrogen cycle: sources and consequences
Terrestrial phosphorus limitation: mechanisms, implications, and nitrogen-phosphorus interactions
DOI:10.1890/08-0127.1 URL [本文引用: 2]
The fate of phosphorus during pedogenesis
DOI:10.1016/0016-7061(76)90066-5 URL [本文引用: 1]
Variations in leaf and root stoichiometry of Nitraria tangutorum along aridity gradients in the Hexi Corridor, northwest China
DOI:10.1134/S1995425514030123 URL
Growth response of Mountain birch to air and soil temperature: Is increasing leaf-nitrogen content an acclimation to lower air temperature
DOI:10.1046/j.1469-8137.2001.00078.x URL [本文引用: 1]
Redistribution of mineral elements during development
DOI:10.1146/arplant.1955.6.issue-1 URL [本文引用: 1]
Nutrient concentrations in plants in the Brookhaven oak-pine forest
DOI:10.2307/1934963 URL [本文引用: 1]
The worldwide leaf economics spectrum
DOI:10.1038/nature02403 URL [本文引用: 3]
The NITREX project: an introduction
DOI:10.1016/0378-1127(94)06080-3 URL [本文引用: 2]
Leaf nitrogen and phosphorus stoichiometry of Quercus species across China
DOI:10.1016/j.foreco.2012.07.025 URL [本文引用: 1]
Reproductive organ and young tissues show constrained elemental composition in Arabidopsis thaliana
DOI:10.1093/aob/mcv190 URL [本文引用: 7]
Effects of nitrogen and phosphorus supply on stoichiometry of six elements in leaves of Arabidopsis thaliana
DOI:10.1093/aob/mcy169 URL [本文引用: 2]
Effects of nitrogen and phosphorus supply on growth rate, leaf stoichiometry, and nutrient resorption of Arabidopsis thaliana
DOI:10.1007/s11104-014-2316-1 URL [本文引用: 6]
Nutrient allocation strategies of woody plants: an approach from the scaling of nitrogen and phosphorus between twig stems and leaves
DOI:10.1038/srep20099 URL [本文引用: 3]
Phosphorus accumulates faster than nitrogen globally in freshwater ecosystems under anthropogenic impacts
DOI:10.1111/ele.2016.19.issue-10 URL [本文引用: 1]
Nutrient addition affects scaling relationship of leaf nitrogen to phosphorus in Arabidopsis thaliana
DOI:10.1111/fec.2018.32.issue-12 URL [本文引用: 10]
An assessment on the uncertainty of the nitrogen to phosphorus ratio as a threshold for nutrient limitation in plants
DOI:10.1093/aob/mcx106 URL [本文引用: 1]
Variations of leaf N and P concentrations in shrubland biomes across northern China: phylogeny, climate, and soil
DOI:10.5194/bg-13-4429-2016 URL [本文引用: 1]
C:N:P stoichiometry of Artemisia species and close relatives across northern China: unravelling effects of climate, soil and taxonomy
DOI:10.1111/1365-2745.12409 URL [本文引用: 3]
A preliminary study on chemical constitutents in Arctic snow
加拿大北极地区雪化学成分的初步研究
Linking stoichiometric homoeostasis with ecosystem structure, functioning and stability
DOI:10.1111/j.1461-0248.2010.01532.x URL [本文引用: 2]
Stoichiometric homeostasis of vascular plants in the Inner Mongolia grassland
DOI:10.1007/s00442-010-1902-z URL [本文引用: 1]
Stoichiometric homeostasis predicts plant species dominance, temporal stability, and responses to global change
DOI:10.1890/14-1897.1 URL [本文引用: 2]
Testing the growth-rate hypothesis in vascular plants with above- and below-ground biomass
DOI:10.1371/journal.pone.0032162 URL [本文引用: 1]
Fine root biomass, production, turnover rates, and nutrient contents in boreal forest ecosystems in relation to species, climate, fertility, and stand age: literature review and meta-analyses
DOI:10.1080/07352689.2010.483579 URL [本文引用: 1]
Global-scale latitudinal patterns of plant fine-root nitrogen and phosphorus
DOI:10.1038/ncomms1346
PMID:21673665
[本文引用: 1]
Most water and essential soil nutrient uptake is carried out by fine roots in plants. It is therefore important to understand the global geographic patterns of fine-root nitrogen and phosphorus cycling. Here, by compiling plant root data from 211 studies in 51 countries, we show that live fine roots have low nitrogen (N) and phosphorus (P), but similar N:P ratios when compared with green leaves. The fine-root N:P ratio differs between biomes and declines exponentially with latitude in roots of all diameter classes. This is in contrast to previous reports of a linear latitudinal decline in green leaf N:P, but consistent with nonlinear declines in leaf litter N:P. Whereas the latitudinal N:P decline in both roots and leaves reflects collective influences of climate, soil age and weathering, differences in the shape of the response function may be a result of their different N and P use strategies.
Decoupling of nitrogen and phosphorus in terrestrial plants associated with global changes
DOI:10.1038/nclimate2549 URL [本文引用: 3]
Nitrogen use efficiency of competing individuals in a dense stand of an annual herb, Chenopodium album
藜个体在高密度种群中的氮素利用效率
DOI:10.17521/cjpe.2004.0043
[本文引用: 1]
氮素利用效率(NUE)是植物养分策略研究中的一项重要内容。该文利用Berendse和Aerts提出的氮素利用效率概念和原理研究了高密度的藜(Chenopodium album)种群中不同植物个体在种内竞争条件下的氮素利用效率。结果表明,由于植株的氮素吸收速率与其个体大小成非线性关系,说明不同植株个体对氮素的竞争属于非对称竞争。个体较大的植株氮素输入较高,而个体较小的植株氮素输出较高,因而较大个体植株的氮素净增加也较高。植株的氮素损失随着个体大小的增加而增加,较大植株个体的氮素浓度随着生长而下降,而较小植株个体的氮素浓度随时间的变化不大,说明个体较小的植株的生长受光照的限制比受氮素的限制更大,而对较大的植株个体而言,它们的生长受氮素的限制更大。高密度藜种群中的不同植物个体具有不同的养分策略,氮素利用效率及其组成部分氮素生产力(NP)和氮素滞留时间(MRT)均不同。植株的NP和MRT与其个体大小正相关,较大的植物个体具有较高的NP和较长的MRT,因而氮素利用效率也高于个体较小的植株。在个体水平上,种内不同植株的NP与MRT不存在权衡关系(Trade-off)。因此,Berendse和Aerts提出的氮素利用效率概念不仅适用于研究种间的养分策略,对于研究种内不同植株的养分策略也同样适用。
Nitrogen resorption from senescing leaves in 28 plant species in a semi-arid region of northern China
DOI:10.1016/j.jaridenv.2005.01.023 URL [本文引用: 1]
Effects of three global change drivers on terrestrial C:N:P stoichiometry: a global synthesis
DOI:10.1111/gcb.13569 URL [本文引用: 2]
Ecological stoichiometry: a science to explore the complexity of living systems
生态化学计量学: 复杂生命系统奥秘的探索
DOI:10.17521/cjpe.2005.0120
[本文引用: 1]
20世纪以来,生物科学的发展异军突起,成为发展最快的学科,不仅学科分类逐渐细化,而且研究领域也逐渐深入,然而,这种分化和深入也可能会掩盖生物的一些最普遍特征。地球上的生物是否具有统一的、更本质的特征?能否把不同生物学领域和不同层次的知识联系起来?随着对这些问题的不断探索,一门新兴的学科——生态化学计量学,在最近20年悄然兴起。生态化学计量学结合了生物学、化学和物理学等基本原理,是研究生物系统能量平衡和多重化学元素(主要是C、N、P)平衡的科学。这一研究领域使得生物学科不同层次(分子、细胞、有机体、种群、生态系统和全球尺度)的研究理论能够有机地统一起来。目前,生态化学计量学已经广泛应用于种群动态、生物体营养动态、微生物营养、寄主_病原关系、生物共生关系、消费者驱动的养分循环、限制性元素的判断、生态系统比较分析和森林演替与衰退及全球C、N、P生物地球化学循环等研究中,并取得了许多研究成果。该文概述了生态化学计量的概念、历史起源和基本理论,重点介绍了生态化学计量学理论在消费者驱动的养分循环、限制性养分元素判别以及全球C、N、P循环等方面的应用进展,并对生态化学计量学未来的研究方向进行了展望,期望引起国内同行的重视并有助于推动我国在此领域开展相关研究。
Nitrogen enrichment alters plant N:P stoichiometry and intensifies phosphorus limitation in a steppe ecosystem
DOI:10.1016/j.envexpbot.2016.10.014 URL [本文引用: 1]
C:N:P stoichiometry in China’s forests: from organs to ecosystems
DOI:10.1111/fec.2018.32.issue-1 URL [本文引用: 1]
Long-term N and P additions alter the scaling of plant nitrogen to phosphorus in a Tibetan alpine meadow
DOI:10.1016/j.scitotenv.2017.12.292 URL [本文引用: 1]
Application of N:P stoichiometry to ecology studies
Resorptions of 10 mineral elements in leaves of desert shrubs and their contrasting responses to aridity
DOI:10.1093/jpe/rty034 URL [本文引用: 1]
Leaf element concentrations of terrestrial plants across China are influenced by taxonomy and the environment
DOI:10.1111/geb.2012.21.issue-8 URL [本文引用: 1]
Intra-annual dynamics of xylem growth in Pinus massoniana submitted to an experimental nitrogen addition in Central China
DOI:10.1093/treephys/tpx079 URL [本文引用: 1]
Root elemental composition in Chinese forests: implications for biogeochemical niche differentiation
DOI:10.1111/fec.2018.32.issue-1 URL [本文引用: 1]
Invariant allometric scaling of nitrogen and phosphorus in leaves, stems, and fine roots of woody plants along an altitudinal gradient
DOI:10.1007/s10265-016-0805-4 URL [本文引用: 5]
Conservative allocation strategy of multiple nutrients among major plant organs: from species to community
DOI:10.1111/jec.v108.1 URL [本文引用: 1]
Nutrient and ecological stoichiometry of different root order fine roots of 59 evergreen and deciduous tree species in subtropical zone
亚热带59个常绿与落叶树种不同根序细根养分及化学计量特征
/
〈 |
|
〉 |
